
Forging Metamaterials: Labs Craft Invisibility Cloaks, Perfect Lenses and Nanostructures (Kavli Roundtable)
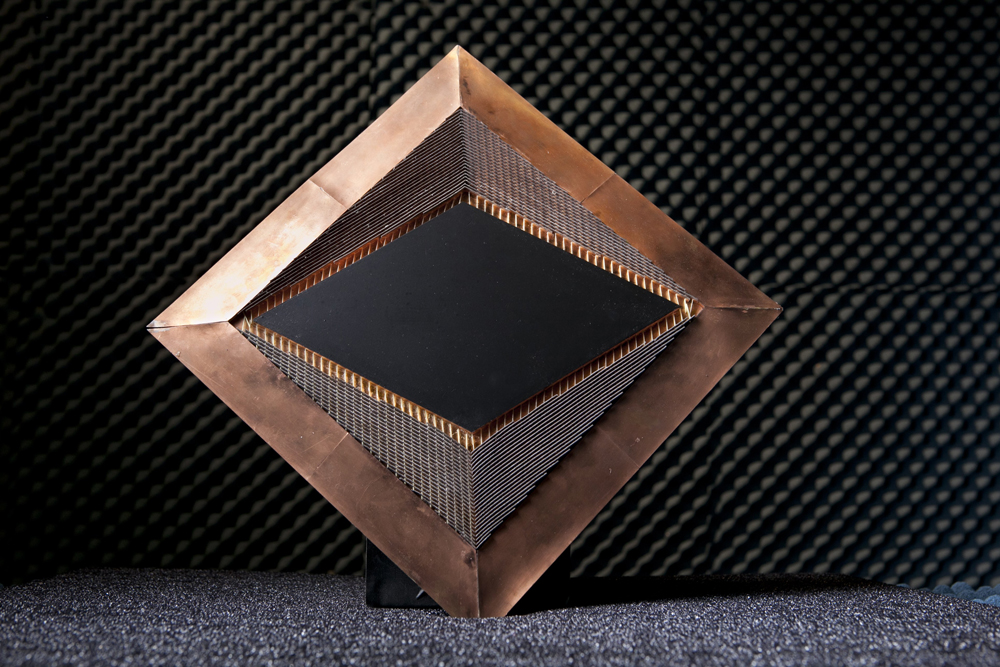
Alan Brown, writer and editor for the Kavli Foundation, edited this roundtable for Live Science's Expert Voices: Op-Ed & Insights.
From bronze alloys to plastic composites, humans have been making artificial materials for thousands of years. Yet metamaterials — conventional materials with unconventional structures that frequently display new and unusual properties — are something entirely different.
By controlling the structure of materials at the nanoscale, scientists and engineers have been able to do things once thought impossible. Such materials have yielded advancements ranging from cloaking devices that render objects nearly invisible to lenses that can see details smaller than what were once assumed to be the fundamental limits of optical resolution.
In fact, researchers have already demonstrated many metamaterials with properties unknown or difficult to achieve in nature, interacting with light and electromagnetic waves in unusual ways, and showing unusual physical behaviors, as well.
Such breakthroughs are only the beginning of what may be possible. To discuss this new class of materials, The Kavli Foundation has brought together three leaders in the field:
Julia Greer, professor of Materials Science and Mechanics and board member of the Kavli Nanoscience Institute at California Institute of Technology. She recently discussed the hierarchical design of metamaterials at a TEDx talk at CERN, Google's Solve for <X> event, and the World Economic Forum in Davos.
David Smith, chair of the Department of Electrical and Computer Engineering and director of the Center of Metamaterials and Integrated Plasmonics at Duke University. Smith demonstrated the first optical metamaterials in 2000, and went on to use metamaterials to create an invisibility cloak. He has also launched several companies based on metamaterial technology. [Science Fiction or Fact: Invisibility Cloaks Will One Day Exist]
Get the Space.com Newsletter
Breaking space news, the latest updates on rocket launches, skywatching events and more!
Xiang Zhang, professor of Mechanical Engineering and member of the Kavli Energy NanoSciences Institute, at the University of California, Berkeley. He is also director of the Materials Sciences Division of the U.S. Department of Energy's Lawrence Berkeley National Laboratory. After developing the first perfect optical lens and pioneering new ways of building nanoscale devices, he developed an acoustic cloak device.
The Kavli Foundation: Some people define metamaterials as materials with structures that do not exist in nature. Is this definition adequate? Does it really capture what makes metamaterials unique?
Julia Greer: I don't think so. People have been struggling because there really isn't a good definition. The three of us on this roundtable clearly highlight this: Xiang knows metamaterials that can mask sound, David knows about metamaterials that can manipulate light, and I know metamaterials that have mechanical properties. The very fact that we have to specify the type of metamaterial tells you that any definition is not as simple as saying it's just something that doesn't exist in nature. Many things don't exist in nature.
My spin on it is that metamaterials are materials whose properties deviate from what would be expected from the same atoms if they were repeated in a normal atomic structure. In that case, we might expect its properties to be one thing. But in these so-called artificial grids of atoms, or lattices, that we've created, those properties are different.
Xiang Zhang: If you look at the Greek definition of "meta," it means something that goes beyond ordinary. It’s important to distinguish between metamaterials and their properties. Metamaterials are indeed built from nature materials. However, they can have properties, such as negative refraction index, that do not exist in nature, and that certainly differ from the parent materials.
Yet this unusual behavior is not limited to just optical or acoustic or structural properties. It could be any property. Their range of properties is very broad.
David Smith: I think it's a mistake to define metamaterials in terms of properties not found in nature, simply because it's really hard to create things that don't exist in nature. Instead, I think the value of metamaterials is that we can design materials so precisely, we can extend their properties beyond what nature provides easily. I think this might be a better definition.
A good example is transformation optics, where we change the material's structure to control the wavelengths with which it will interact. These precise, controlled structures enable us to create material properties on demand. There are a lot of ways to accomplish this, but the goal is to obtain precisely the properties you want by creating structures that were either impossible or difficult to achieve before.
J.G.: May I just add one more thing? I think that the "meta" part emerges when we get into the nanoscale realm. This is when the dimensions within the structure approach the dimensions of whatever energy is exciting it. In photonic metamaterials, for example, the structures are comparable in size to the wavelength of light they interact with. In my mechanical metamaterials, the repeating structures approach the scales of the links that hold the materials together. Whenever the lattice's critical features are small enough to interact with whatever excites it, that's when you start seeing these very interesting effects.
TKF: So are metamaterials all about size?
J.G.: It's not only size. For us, it's very much the combination of size, the material, and the material's geometry, its structure. What makes metamaterials unique is that these aspects are not independent of one another anymore.
For example, metamaterials have unique properties because they interact with forces at the nanoscale in ways that we can only explain by using quantum mechanics. If we scaled those structures up to a meter or a kilometer, they would no longer exhibit the same properties. At that size, we could describe their properties using conventional structural engineering and material science.
In a metamaterial, the material, structure, and properties are coupled together in ways that produce unexpected results. Size is a huge component of this, but only because it's linked with material composition and structure.
TKF: Does everyone agree? Must metamaterials be small enough to interact with the waves and energy around them?
D.S.: We're really talking about two different regimes. Some of the first work on metamaterials started after we began modifying photonic crystals, which are the optical analogs of semiconductors. The spacing of elements on photonic is typically on the order of the wavelength you want to work with.
In metamaterials, there is another, smaller regime. Roger Walser, who coined the term "metamaterials," thought we could structure magnetic materials in new ways to handle heat better and improve other properties. His idea was to make elements much smaller than the excitations we were trying to manage.
When our group talks about metamaterials, we generally mean elements that are much, much smaller than the wavelengths we want to work with.
X.Z.: That is true in our group, as well. Historically, metamaterials referred to materials with repeating periodic building blocks called “unit cells” that were smaller than the wavelength of electromagnetic, acoustic, or other waves we wanted to manipulate. That is still the case for Dave and me. In a sense, we are creating mixtures. But instead of getting properties that reflect the amount of materials we combine randomly, the properties of metamaterials can be very different from simply averaging the properties of the starting materials.
J.G.: That's a really good point. There's also another distinction between metamaterials. One type interacts with some type of wave. The wave passes through it, but doesn't damage the metamaterial in any way. Then there are structural metamaterials, where some type of force deforms the material or changes its properties. From a distance, the material looks like any other homogeneous material, but then it exhibits properties that you would never anticipate.
TKF: So let me change the subject for a moment. I recently looked up references to "metamaterial" on Google Scholar. In 2000, researchers published only 49 papers that used that term. In 2014, that number jumped to more than 10,000 papers.
D.S.: Wow.
TKF: What spurred this phenomenal growth, and what does it say about how the field is changing?
D.S.: Several things started to come together in 2000. John Pendry, who recently won the Kavli Prize in Nanoscience for his work on metamaterials, had predicted that an artificially structured material called a split-ring resonator would produce a magnetic response. While trying to verify that, we created a left-handed material with a negative index of refraction.
TKF: Could you explain what a left-handed material is?
D.S.: It is a material that has properties that are the opposite of what you would expect from a conventional, or natural, right-handed material. So a left-handed negative refraction index material would bend light in the opposite direction of a conventional material.
We really didn't know anything about negative index materials when we started doing these experiments. But negative refractive index materials have many other properties that interested physicists. For example, they also reverse Doppler shift and Cherenkov radiation.
At the same time, the Defense Advanced Research Projects Agency, or DARPA, was calling for proposals to create structured materials with properties not found in nature. Our negative index material certainly fit the description, and showed what could be done. It became a poster child for a metamaterial.
John Pendry, meanwhile, started wondering how we might build on it. He came up with this idea of the perfect lens, which used its negative index to achieve much higher resolution than was previously thought possible. He published his paper several months later. That paper came to define the field, because it was such a counterintuitive prediction, that you could actually create a lens that entirely surpassed what everyone had previously believed was the theoretical limit of optical resolution.
Of course, there are lots of caveats that I'm not including, all the years and years of discussion and debate. Xiang, in fact, actually did the experiment that pretty much convinced everyone that the things we were seeing from negative index materials were real.
Later, our team used metamaterials to create an invisibility cloak. Xiang used them to create a device that cloaked sound. These were all big hits because they showed interesting effects and physics that you just can't do with conventional materials. That really drove the field, the ability to start from scratch and create a material to get the physics you're looking for.
TKF: Xiang, could you tell us about that experiment?
X.Z.: John Pendry's theoretical work made two predictions. One was the negative index of refraction, which David demonstrated with microwaves. The second involved imaging objects smaller than a couple of hundred nanometers. In conventional lenses, those objects are smaller than the wavelength of visible light, so we cannot use visible light to pick out their details. We showed that Professor Pendry's theory indeed works, and that we could image objects smaller than that limit using a perfect lens.
It's an exciting time in our field. People are working on acoustic and thermal properties, and Julia and others are working on structural properties. John Pendry's work is really exciting, and there have been many surprises along the way.
J.G.: I think some researchers have learned that if they use "metamaterial" to describe their work, they will have higher visibility with funding agencies and journals. But the real reason for our field's growing interest is that it lets us access so much untapped territory. As David and Xiang said, there's a wealth of information emerging about so many different phenomena. In some cases, we already know what we're looking for, but in many cases, we don't really know what to expect. For example, we never expected our ceramic nanolattice to recover from compression the way it did.
TKF: You're referring to a recent experiment. You built a three-dimensional lattice out of thin ceramic tubes, crushed it in a vise, and it sprang back to shape, right? You didn't expect that to happen?
J.G.: Oh, absolutely not. There was no way. We were really shocked. We actually have a video now where our instrument malfunctioned. Instead of stopping where it should have, it just squashed the heck out of the nanolattice. You would expect the lattice to be super, super damaged. And, sure, little bits of it flew off. But when we removed the load, it recovered and bounced back to its original shape.
These were real effects that nobody could have predicted before, and that's exciting. We call them "metamaterials" for the lack of a better word, but what exactly are they? They are materials that have all these unexpected effects and properties. The reason why, at least in our world, is the very intricate interplay between material composition and structure at nanoscale dimensions. You would never see these effects anywhere else. As researchers learn to build these structures, we are discovering more and more phenomena. There's so much more that we can learn, and it makes perfect sense for this field to be exploding.
Also, metamaterials are teaching us that we are no longer slaves to coupled properties. In my world, for example, density has always been coupled to every single mechanical attribute. So lightweight materials like aluminum have always been weaker than denser materials like steel. What our work showed, to pick one example, is that you can reduce weight by several orders of magnitude while retaining exactly the same strength. There is no coupling of density and strength. So, it really shifts the way we think about materials.
TKF: Clearly, we are learning a lot about metamaterials. So, do we understand the theories behind them well enough to create materials with the properties we want?
J.G.: We're a couple of years away from that, at least in the mechanical materials we work with. We know a lot about materials properties. What we need is a tool that tells us that if we want this strength and density, we use this material and structure. That tool doesn't exist today. But we're getting closer to populating the library of information necessary to make those predictions.
D.S.: On the electromagnetic side, it's a pretty solved problem. If we want certain properties, we know how to get them. Everything has been really perfectly predictable since 2000, because that was what we had to do to make negative index materials and transformation optics, which really need perfect structures. On the acoustics side, my colleague at Duke, Steve Cummer, and Xiang, can also predict the structures they need.
X.Z.: Yes, one can predict the properties for a given structure once it is designed. There is, however, another side of the story. This involves exploring really unknown properties, things that were not possible before. Negative index metamaterials fell into that category once.
Another example involves molecules with unusual symmetries. Ordinarily, the thermodynamics of a chemical reaction drive it to produce molecules with certain types of symmetries. For example, two molecules may bond end-to-end or side-to-side. Chemists have tried to synthesize molecules to show other types of symmetry for centuries — for example, twisted ring, inside-out Mobius symmetry — but failed. We have found a way to do that, but it required a great deal of design intuition. The tough question is, can we find the way to design the unit cell for a desired properties?
TKF: So, your design starts with a basic repeating unit, a unit cell. As we discussed before, most metamaterials consist of these repeating structures. But the metamaterials Julia works with are hierarchical, that is, the structures start at the nanoscale and change as they grow progressively larger.
J.G.: Yes. Nature builds hierarchy into its hard materials, such as seashells, crab shells and even such primitive organisms as diatoms. The ocean bangs them around and subjects them to extreme temperatures and pressures, yet they don't break. But if you think about it, they're 99 percent ceramic. They should break, like glass. So there's something about the construction of these materials that makes them particularly damage tolerant.
Their design is very clever. Some use a soft protein to glue their ceramic platelets together and absorb shock. Others use air. Diatoms, for example, are made out of silica - glass - with holes in it. If you throw a glass bottle into the ocean, it will break and the sand with smooth its edges. But diatoms don't break apart, and they retain their sharp features.
Researchers often wave their hands and say that the reason biomaterial properties are so amplified is because of hierarchy, but there are many competing explanations for why this is so. So we've tried building hierarchical structures.
An example is the structures that we crushed and then sprang back. We started with hollow ceramic nanotubes that formed triangular nanotrusses that look like a gantry on a giant crane. Then we form those nanotrusses into a second order structure, a nanolattice of nanotrusses.
Adding hierarchy makes them a lot more damage tolerant. It becomes nearly impossible to break it. If you were to make paper out of them, it would be completely tear-proof. It's really cool, like holding a piece of paper that's made out of a cloud that's really light but you can't tear it apart. Why? Because those hierarchical elements localize damage or defects in the structure, so they can't spread.
TKF: Xiang, you think about hierarchies differently, don't you?
X.Z.: Nature also shows us how structure shapes properties. If we start with carbon atoms, we can form very hard diamonds or graphite that is soft enough to rub off when we use it in pencils. It's the same atom, but the architecture, the geometry of atoms, gives us totally different properties.
Julia's ideas about hierarchical structures lie beyond that. I don't think many people who have studied electromagnetic, optical, or acoustic metamaterials have looked at hierarchies that way. But some are exploring the fractal structures, which are structures that repeat at different scales, for antenna and other things.
TKF: So, let's switch gears. So far, we've been talking about laboratory experiments. How far are we from commercializing products based on metamaterials?
D.S.: It depends on which area we're talking about. We found huge opportunities for metamaterials in microwave devices. That's led to three spinoff companies. Kymeta Corporation is probably the best known, and is dedicated to satellite communications. There is also Evolv Technology, which does security imaging, and Echodyne, which is involved in radar. All three are commercializing metamaterial concepts, and all address product areas that have unmet needs, so it's kind of an exciting time for the commercialization.
TKF: Kymeta is the furthest along. Could you tell us about that?
D.S.: It is gearing up for large-scale production of antennas for satellite communications. In the past, earth stations could only communicate with satellites in a few ways. You could use small, stationary dishes aimed at geosynchronous satellites, which keep the same position in the sky. Or you could build large dishes to track moving satellites, but they are expensive and have all the problems associated with really massive physical systems. A third approach, phase array systems, is incredibly expensive and burns a tremendous amount of power.
Using metamaterials and some other technologies, Kymeta is introducing a low-cost antenna that we can steer electronically so it can track moving satellites. It really opens up the possibility of ubiquitous, high bandwidth satellite communications. [Nanotechnology's Big Impact ]
TKF: How do metamaterials make this possible?
D.S.: It turns out that metamaterials are relatively easy to tune because they resonate. So we apply a metamaterial over a layer of electronics, then use the electronics to tune the resonance of the metamaterials.
The antenna itself is a cross-disciplinary mix of material concepts, antenna concepts, and engineering. The metamaterials give us a way to unite several different technologies and leverage them to create this low-cost alternative. The result is a steerable antenna that can track any satellite we want, even from moving cars or airplanes.
That's the type of opportunity that's out there. It's easiest to address in the microwave regime, but I think we'll see the same type of thing for infrared, visible, and terahertz waves over the next few years, and also in other spaces like acoustics and structural materials.
TKF: Xiang, you mentioned a process to make metamaterials with unusual symmetries. You can do this in bulk, which is a first step towards commercialization. Could you tell us about the materials and how you might use them?
X.Z.: As Dave mentioned, making negative refractive index materials is still hard, especially ones that work in all three dimensions. This is a challenge we've been working on, low cost, high volume, 3D metamaterials.
For example, we are trying to make negative index materials by self-assembly. This is a potentially economical way of making lots of these materials. But the thermodynamics of self-assembly usually gives you very symmetrical materials. But if you want metamaterials with really unique properties, especially in all three dimensions, they need to have less symmetry. So what we've done is develop a self- assembly process with a self-correction mechanism. We do this with particles suspended in liquid. When the desired geometry is right, they will bond together. If not, they will destroy themselves.
TKF: Once you've made these materials, are they useful?
X.Z.: Yes, of course. We can use metamaterials with a negative index of refraction to control optical beams, or make a lens that can image small things, such as a virus, with super resolution. These devices can focus laser beams down to nanometer scale, making the interface with nanoscale electronics much easier. Bridging that size difference makes these devices very powerful. That is something really unique. In fact, we are using these materials to design microwave devices that can manipulate data in quantum networks in ways that will drastically improve efficiency.
TKF: What about you, Julia. How far are we from scaling up in mechanical systems?
J.G.: Our world is struggling with this right now. We use a process called two-photon lithography, to produce very small structures, maybe 100-micron cubes, from lager pieces of material. If we want them to be useful, we have to scale up the number of devices we produce, rather than trying to produce larger device.
Unfortunately, two-photon lithography is great at creating very fine nanostructures, but it is not amenable to scaling up at all. Some people have suggested a technique called interference lithography, which carves small things by using the interference patterns created by two beams of light. It can't generate features that are as complex as the ones we make with two-photon lithography.
Also, interference lithography is generally used to make two-dimensional objects. That's fine for patterning surfaces that interact with electromagnetic waves. But for structural materials, we need to build in three dimensions because they need to interact with physical forces. To do that, we need tools that will enable us to create hierarchical systems from the nanoscale on up. The National Science Foundation recently issued a call for new types of equipment, so we will see if there are other promising technologies out there.
TKF: We've been talking about commercializing metamaterials. Now I would like you to step back for a moment and look at the big picture. What do you see as the grand challenges in metamaterials?
J.G.: Scalability. That is the major roadblock right now. The limiting factor is writing the initial structure, and that's what we're aggressively pursuing in my group right now.
D.S.: I'm always bad with these grand challenges things. Instead, we move from one interesting thing to the next. But I would say that our ongoing challenge is to identify potentially interesting research areas that we can transition to the applied world.
It's really a question of marketing. There are lots of things that are really interesting but don't have a chance of making it to market because they either have no real application or they will never be practical. So, we put a lot of effort into trying to find uses for potential metamaterial devices, because without some commercially compelling applications, the field will start to decline.
TKF: Spoken like an engineer.
D.S.: And to think, I started out as a physicist.
TKF: Xiang, how about you?
X.Z.: We certainly see lots of challenges. We want to make materials that have unusual properties in more than one dimension in quantities large enough for practical applications.
There is also a huge interest in using metamaterials to create a major impact in other fields, such as imaging or quantum information. For example, we are working with a research group in Austria to validate a theory about quantum properties that we could not test in the past. We may or may not succeed, but I think we could use metamaterials to create new experimental platforms to test many interesting theories. There are tons of possibilities.
Follow all of the Expert Voices issues and debates — and become part of the discussion — on Facebook, Twitter and Google+. The views expressed are those of the author and do not necessarily reflect the views of the publisher. This version of the article was originally published on Space.com.
Join our Space Forums to keep talking space on the latest missions, night sky and more! And if you have a news tip, correction or comment, let us know at: community@space.com.