What are pulsars?
These 'cosmic lighthouses' can spin as fast as 700 rotations per second.
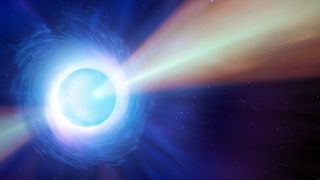
Pulsars are rapidly rotating neutron stars that blast out pulses of radiation at regular intervals ranging from seconds to milliseconds.
Pulsars have strong magnetic fields that funnel particles along their magnetic poles accelerating them to relativistic speeds, which produces two powerful beams of light, one from each pole. According to the University of California, because the poles of the magnetic field aren't aligned with the axis of spin of the pulsar, the beams of particles and the light they produce are swept around as the pulsar rotates.
The periodicity of pulsars is caused by these beams of light crossing the line of sight here on Earth, with the pulsar appearing to 'switch off' at points when the light is facing away from us. The time between these pulses is the 'period' of the pulsar.
Related: How many stars are in the universe?
Imagine this as a pair of open scissors spinning on one handle. One blade points upwards, this is the axis of rotation, while the other blade is the beam of light. While the axis of rotation has the same orientation, the direction of the beam turns with the neutron star's spin.
Thus, in effect, pulsars can be thought of as 'cosmic lighthouses.' Even though the lighthouse may be sending out beams of light all night but stricken sailors only see it when it is facing toward them. Because they spin so rapidly this takes the appearance of 'flickering.'
This also means that the name 'pulsar' may actually be misleading as these neutron stars don't actually pulse by periodically shrinking and swelling in size, as astronomers once thought. Their pulsing is merely a factor of their orientation in relation to our view of them, their light output is mostly consistent.
There are two types of pulsars, ones with periods of a few milliseconds with this periodicity changing very slowly over time, called millisecond pulsars, and other pulsars which are just called 'ordinary pulsars.'
How do pulsars form?
Like all neutron stars, pulsars are born when stars with masses between four and eight times that of the sun run out of fuel for nuclear fusion, says Penn State University. When the fusion of lighter elements into heavier elements stops, the production of energy that supports the massive star against the inward pressure of its own tremendous gravity also ceases. The balance the star has enjoyed all of its life ends and it begins to collapse.
As the collapse proceeds the outer layers of the star are blown away in a supernova explosion with only the iron core of the massive star containing masses equivalent to that of the sun up to about 1.5 times that of our star remaining. This crushes down into a width no greater than around 12 to 17 miles (19 to 27 kilometers), around that of a city here on Earth, according to NASA Goddard.
This creates neutron star matter composed of 95% neutrons, because the collapse has forced electrons and protons together. According to NASA, the material that comprises neutron stars is so dense that a mere teaspoon of it would weigh 4 billion tons. This is equivalent to 10,000 Empire State Buildings stacked on a tiny spoon!
This superdense material is prevented from cramming further together as the mass of the stellar core cannot overcome the quantum properties of its neutrons. If the star was massive enough to overwhelm this effect the neutron star would continue to collapse until it transforms into a black hole.
A star with the mass of the sun will never become a neutron star, instead, our star will end its life having run out of hydrogen to fuse into helium as a smoldering stellar remnant called a white dwarf.
The process of core collapse may be what causes young neutron stars to spin rapidly as pulsars. Think of this as akin to an ice skater drawing in their arms. As they do so, the ice skater spins more rapidly. This is analogous to the rapidly shrinking diameter of a collapsing stellar core.
One theory as to why millisecond pulsars rotate even more rapidly is that they are born from massive stars in a binary system. After the neutron star creation process has concluded the newborn neutron star strips material from its close binary companion. This transfers angular momentum from the doner star to the 'feeding' neutron star which increases its rotation or 'spins it up.'
Are all neutron stars pulsars?
In short, all pulsars are neutron stars but, not all neutron stars are pulsars.
The majority of neutron stars we have discovered thus far are pulsars, but that's because they are far more obvious than neutron stars. With large beacons of radiation blasting out from magnetic poles, astronomers can observe these cosmic lighthouses far easier than small, dim, neutron stars.
However, some pulsars may not be observable from Earth, because their radiated beams of light don't ever orientate themselves toward us.
We can be fairly certain that pulsars are rapidly spinning neutron stars because young pulsars have been detected within the remnants of supernovas, exactly where neutron stars are expected to be found.
Other neutron stars that currently don't appear to be pulsars and look like non-rotating neutron stars, may have once been pulsars but the process that causes them to blast out beams of radiation may have 'turned off' or the emissions may be too weak to be observed. The average lifetime of a pulsar is around 10 million years and as they age their rotation slows.
So if not all neutron stars are pulsars how do some of these exotic remnants end up like powerful cosmic lighthouses?
Who discovered pulsars?
Following the discovery of neutrons in 1932 by English physicist James Chadwick, the concept of a neutron star was first predicted in 1934 by Lev Landau in the Soviet Union and separately by Walter Baade and Fritz Zwicky in the U.S. during the same year.
Five years later in 1939, Robert Oppenheimer and George Volkoff would develop a theoretical model for neutron stars, but it would take a further three decades for the first neutron star, in the form of a pulsar to be discovered.
In 1967 radio astronomers in Cambridge were involved in the search for quasars and had developed an instrument capable of detecting rapid and random changes in the intensity of radio waves that saved such signals for later analysis.
Examining this data a then 24-year-old graduate student at New hall, conducting research at Cambridge's Cavendish Laboratory, Jocelyn Bell under the supervision of Antony Hewish, discovered mysterious highly regular signals very different from the random signals expected from quasars affected by solar winds.
The signals were so regular that when they were first discovered they were attributed to human activity. When it was deduced that these highly regular signals must originate from the universe, the suggestion was made that they could be the result of intelligent extraterrestrial life.
This led to the source of these pulses earning the tongue-in-cheek name 'LGM1' or 'Little Green Man 1.' Of course, Bell and Hewish went on to discover the actual source of the emission was a pulsar with a period of 1.3373 seconds. This pulsar now has the slightly less sensational name of PSR B1919+21.
The University of Cambridge says that as of today over 3,000 pulsars have been discovered and while these were initially found in radio waves, we have since discovered these cosmic lighthouses in X-ray, gamma-ray, and even visible light.
Pulsar extremes: How fast can pulsars rotate?
Like all neutron stars, pulsars have quite tightly constrained masses and sizes, most neutron stars have a mass of around 1.5 times that of the sun. One respect in which pulsars vary a lot is their rate of rotation. Some spin at a rate of hundreds of times per second, which NASA says is faster than the blades of a household blender.
In 2022 astronomers discovered the heaviest neutron star to date with a mass of 2.35 times that of the sun, which is also the fastest-rotating pulsar ever found in the Milky Way. The pulsar designated PSR J0952−0607 is also known as the Black Widow pulsar as it is believed to have reached record-breaking rotational speed and mass by consuming a companion binary star.
The Black Widow pulsar rotates at 707 Hertz (HZ), or 707 times per second. This is topped by the pulsar PSR J1748–2446ad which rotates at 716 HZ or 716 times per second.
In 1974, Russell Alan Hulse and Joseph Hooton Taylor Jr., of the University of Massachusetts Amherst discovered another extreme type of pulsar, one existing in a binary system with a neutron star.
Named a Hulse–Taylor binary, this type of pulsar system has become extremely important to astronomers looking to study the limits of Albert Einstein's theory of general relativity and for the study of gravitational waves, tiny ripples in the very fabric of spacetime that propagate from the universe's most powerful and violent events and objects.
Related: Mysterious pulsar spins too slowly with 7 different pulse patterns
Uses of pulsars
Pulsars are fantastic cosmic tools for scientists to study a wide range of phenomena.
The light emitted by a pulsar carries information about these objects and what is happening inside them. That means pulsars give scientists information about the physics of neutron stars, which are the densest material in the universe (with the exception of whatever happens to matter inside a black hole). Under such incredible pressure, matter behaves in ways not seen before in any other environment in the universe. The strange state of matter inside neutron stars is what scientists call 'nuclear pasta': Sometimes, the atoms arrange themselves in flat sheets, like lasagna, or spirals like fusilli, or small nuggets like gnocchi.
Some pulsars also prove extremely useful because of the precision of their pulses. There are many known pulsars that blink with such precise regularity; they are considered the most accurate natural clocks in the universe. As a result, scientists can watch for changes in a pulsar's blinking that could indicate something happening in the space nearby.
It was with this method that scientists began to identify the presence of alien planets orbiting these dense objects. In fact, the first planet outside Earth's solar system ever found was orbiting a pulsar.
Because pulsars are moving through space while also blinking a regular number of times per second, scientists can use many pulsars to calculate cosmic distances. The changing position of the pulsar means the light it emits takes more or less time to reach Earth. Thanks to the exquisite timing of the pulses, scientists have made some of the most accurate distance measurements of cosmic objects.
Pulsars have been used to test aspects of Albert Einstein's theory of general relativity, such as the universal force of gravity.
The regular timing of pulsars also may be disrupted by gravitational waves — the ripples in space-time predicted by Einstein and directly detected for the first time in February 2016. There are multiple experiments currently searching for gravitational waves via this pulsar method.
Using pulsars for these types of applications depends on how settled they are in their rotation (thus providing very regular blinks), Scott Ransom, a staff astronomer at the National Radio Astronomy Observatory (NRAO) in Charlottesville, Virginia said.
All pulsars are slowing down gradually as they spin; but those used for precision measurements are slowing down at an incredibly slow rate, so scientists can still use them as stable time-keeping devices.
Pulsar graveyards
All pulsars slow down gradually as they age. The radiation emitted by a pulsar is jointly powered by its magnetic field and its spin. As a result, a pulsar that slows down also loses power, and gradually stops emitting radiation (or at least, it stops emitting enough radiation for telescopes to detect), Harding said. Observations thus far suggest that pulsars drop below the detection threshold with gamma rays before radio waves. When pulsars reach this stage of life, they enter what's known as the pulsar graveyard. (Pulsars that have stopped emitting may be considered ordinary neutron stars by astronomers).
When a pulsar forms from the wreckage of a supernova, it spins fast and radiates a lot of energy, Ransom said. The well-studied Crab Pulsar is an example of such a young pulsar. This phase may last for a few hundred thousand years, after which the pulsar begins to slow down and only emit radio waves. These 'middle-age' pulsars likely make up most of the population of pulsars identified as emitting only radio waves, he added. These pulsars live for tens of millions of years before eventually slowing down so much that they 'die' and enter the pulsar graveyard.
But if the pulsar sits near a stellar companion, it may be 'recycled,' meaning it siphons material and energy from its neighbor, increasing its spin to hundreds of times per second — thus creating a millisecond pulsar, and giving the once-dead pulsar new life. This change can occur at any time in a pulsar's life, meaning a 'dying' pulsar's rotation rate can increase over hundreds to millions of years. The pulsar begins to emit X-rays, and the pair of objects is known as a 'low-mass X-ray binary,' Ransom said. (These cannibalistic pulsars have been called 'black widow' pulsars or 'redback' pulsars in reference to two species of spiders that are known to kill their companions). Millisecond pulsars are the oldest known pulsars — some are billions of years old and will continue to spin at those high rates for billions of years.
Additional resources
Pulsars aren't the only extreme example of neutron stars in the universe. Magnetars are a type of neutron star that are thought to have the most powerful magnetic fields in the universe. You can read more about them with these resources from NASA. Learn more about pulsars with these learning assets from the online educational website study.com.
Follow us on Twitter @Spacedotcom, and on Facebook.
Bibliography
Neutron stars, Imagine the Universe, NASA, [Accessed 01/14/23][https://imagine.gsfc.nasa.gov/science/objects/neutron_stars1.html.]
Pulsars, Encyclopedia Britannica, [Accessed 01/14/23], [https://www.britannica.com/science/pulsar]
An Introduction to Pulsars, CSIRO, [Accessed 01/14/23], [https://www.atnf.csiro.au/outreach/education/everyone/pulsars/index.html]
S. G. Ryan., A. J. Norton., Stellar Evolution and Nucleosynthesis, Cambridge University Press, [2010], IBSN-978–0–521–13320–3
S.F. Green., M. H. Jones., An Introduction to the Sun and Stars, Cambridge University Press, [2010], IBSN-978–1–107–49263–9
U. Kolb., Extreme Environment Astrophysics, Cambridge University Press, [2010], IBSN-978–0–521–18785–5
NASA Continues to Study Pulsars, 50 Years After Their Chance Discovery, NASA TV, [2017], [https://www.nasa.gov/feature/goddard/2017/nasa-continues-to-study-pulsars-50-years-after-their-chance-discovery]
R. W. Romani., D. Kandai., A, V. Filipenko., et al, PSR J0952−0607: The Fastest and Heaviest Known Galactic Neutron Star, The Astrophysical Journal Letters, [2022], [https://iopscience.iop.org/article/10.3847/2041-8213/ac8007]
Join our Space Forums to keep talking space on the latest missions, night sky and more! And if you have a news tip, correction or comment, let us know at: community@space.com.
Get the Space.com Newsletter
Breaking space news, the latest updates on rocket launches, skywatching events and more!
Robert Lea is a science journalist in the U.K. whose articles have been published in Physics World, New Scientist, Astronomy Magazine, All About Space, Newsweek and ZME Science. He also writes about science communication for Elsevier and the European Journal of Physics. Rob holds a bachelor of science degree in physics and astronomy from the U.K.’s Open University. Follow him on Twitter @sciencef1rst.
- Calla CofieldSenior Writer