Cosmic Clocks Keep Time in the Search for Gravitational Waves
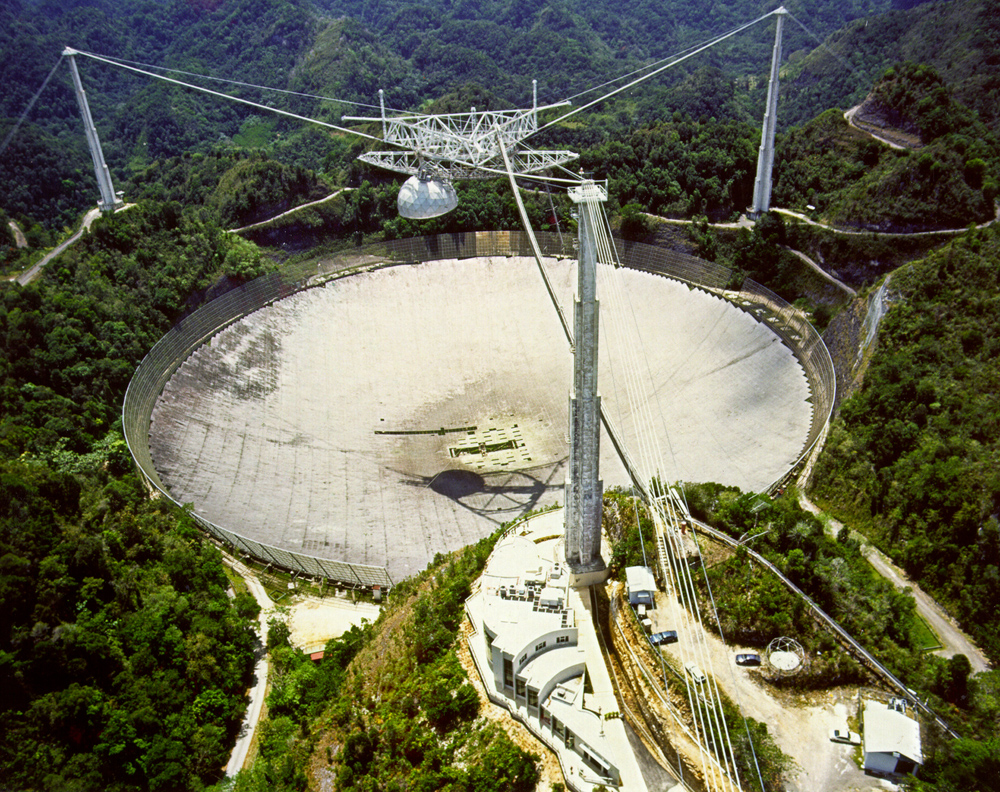
There's a recurring plot line in science fiction and fantasy stories about clocks that start behaving strangely: In "The X-Files," digital clocks go haywire and wristwatches show missing time during alien encounters; in "Doctor Who" and "The Magicians," a clock behaving strangely signals the arrival of a threatening intruder.
With cosmic clocks, an interruption could mean the passing of a ripple in the fabric of space and time.
The clocks in this case are pulsars — objects in the sky that appear to blink rapidly, such as strobe lights. A group of scientists spread out around the world are keeping a careful watch on a handful of pulsars to try to detect those ripples in the fabric of reality, also known as gravitational waves. These pulsar experiments require an incredible amount of patience; some of them have been collecting data for over a decade, and optimistic predictions say it could be another three to five years before they find what they're looking for. [Cosmic Clocks Help Search for Gravitational Waves (Video)]
Lighthouses in the sky
In February, a U.S.-based experiment known as the Laser Interferometer Gravitational-Wave Observatory (LIGO) made history when it announced the first-ever direct detection of gravitational waves. In June, LIGO announced a second detection, and in what seemed like the blink of an eye, the experiment transformed gravitational-wave astronomy from hypothetical to concrete. Scientists can now use gravitational waves to see powerful and previously invisible cosmic events, such as isolated black holes colliding in space.
But LIGO isn't the only instrument hunting for these space-time ripples. Just as there are telescopes that search for different wavelengths of light in the universe (such as X-rays, gamma- rays and radio waves), there are searches aimed at finding different frequencies of gravitational waves; those different frequencies would illuminate different objects and events in the universe.
Pulsars are a subgroup of objects called neutron stars — dense little nuggets of material left over after a star dies. Most neutron stars don't radiate much light, but pulsars are like cosmic lighthouses, simultaneously rotating and radiating dual beams of light. As the beams swing past Earth again and again, astronomers on the ground see a pulse of light. The fastest pulsars spin so quickly that they appear to pulse hundreds of times per second. (Once again, that means something the size of New York City, with more mass than the sun, can spin on its axis hundreds of times per second. The speed and energy involved is mind-boggling.)
But what truly astounded pulsar scientists over the years was the discovery that in some of these fast pulsars, those pulses are more accurately spaced than the second hand on a wristwatch; and some pulsars are so precise that they rival the most accurate clocks ever built by humans. In a universe where so many things seem disordered and messy (even if they obey a strict set of physical laws), the neatness of pulsar timing is striking. (The scientists who first discovered pulsars were so surprised by the regularity of the blinking, they couldn't help but consider that these were signals from an intelligent alien civilization).
Get the Space.com Newsletter
Breaking space news, the latest updates on rocket launches, skywatching events and more!
And so it is understandably strange to see the pulses of light from a very accurate pulsar suddenly not be so accurate. A change in the arrival time of these pulses suggests that something is happening as the light travels toward the Earth; perhaps a gravitational wave passed by, and stretched or compressed the space between the pulsar and the Earth. And that's what scientists around the world are hunting for with Pulsar Timing Arrays.
A treasure trove
Albert Einstein coined the term "gravitational wave" a century ago to describe ripples in the flexible universal fabric he called "space-time." Massive objects create curves in space-time, such as how a bowling ball curves a mattress when placed on it. Two massive objects rapidly spinning around each other not only curve space-time, but also create waves that spread out into the universe, like ripples spreading across the surface of a pond.
Detecting gravitational waves is like searching for boats on the ocean by looking at the waves that crash onto the shore. What would it take to read those waves, and be able to figure out that a boat had passed by somewhere beyond the horizon? What would it take to determine the size of the boat, and the speed at which it was moving?
The LIGO experiment uses an instrument called an interferometer, which directly observes the stretching and compressing of space caused by gravitational waves. LIGO spotted two gravitational-wave events last year — in both cases, the space-time ripples came from black holes, each between about 10 and 40 times the mass of the sun, in the last few seconds before they collided and merged.
Pulsar Timing Arrays, on the other hand, are sensitive to merging black holes that are much, much larger than those seen by LIGO — hundreds to billions of times the sun's mass, according to Maura McLaughlin, a professor of astrophysics at West Virginia University. McLaughlin is a member of Nanograv, a U.S. and Canadian PTA collaboration.
While an object with the mass of the sun can have enough gravitational oomph to cause planets to orbit around it, these supermassive black holes can influence the motions of billions of stars. If or when PTAs reveal two monster black holes merging, it will be at the center of two galaxies that have also merged together, in a process that could be fairly common in the universe.
"While LIGO has probed, in an amazing way, stellar evolution and the fundamental properties of black holes, what Nanograv will be probing is galactic evolution, and galaxy mergers, and cosmology — we'll be looking very far back in cosmic time," McLaughlin said during a talk at the 2016 April meeting of the American Physical Society in Salt Lake City. "So we'll be studying very different things."
Besides seeing black hole mergers, PTA's wills search for a universal gravitational-wave background: a consistent hum of space-time ripples from all the universe's merging, colliding, coalescing action. That background, depending on how strong it is, could be the first thing PTA's are able to detect, McLaughlin said.
Standing on the shore
If scientists want to use pulsars to detect passing gravitational waves, they first have to subtract all the other things that could affect the arrival time of the regular light pulses as they travel to Earth. Things like the motion of the Earth around the sun (if the distance between the pulsar and the Earth changes, then it will take the pulses slightly more or less time to reach the detectors). There could be a companion star close to the pulsar and this introduced a "ton of new things" to account for, McLaughlin told Space.com, such as causing the pulsar to move in an orbit around the companion (further changing how it moves relative to Earth), or the possibility that the gravity of the second object warps the path of the light coming from the pulsar. Just about every variable has a unique answer for every pulsar, and the PTAs have dozens of pulsars that they monitor. McLaughlin's naturally positive tone never falters when she says that after more than 40 years of studying these objects, pulsar scientists are quite comfortable with the process of subtracting these effects.
"It's the name of the game," she said during her talk at the APS meeting. At the same time, she adds that pulsar scientists are constantly revisiting their lists of possible interruptions, making sure they've considered every possible effect. "We're always trying to do better," she said.
PTAs also differ significantly from LIGO in the timing of their gravitational-wave detections. Before black holes collide, they circle each other like water swirling around a drain. LIGO collects gravitational waves created in the last few seconds before the merger. But the massive black holes that PTAs hope to see will still be a long way from actually colliding; many of them not for millions of years, McLaughlin said. That's partly a good thing because it means there's a larger window of time in which a PTA could spot a gravitational-wave event. (Whereas LIGO's window is only a few seconds long, meaning if the detector is turned off when the wave passes by, it won't see it.)
But it also means Nanograv has to collect data over a longer period in order to see the gravitational-wave signal emerge, and after more than 11 years of observation, Nanograv has yet to discern a gravitational-wave signal. (Nanograv's latest data set will be released by the end of the year). When a gravitational wave from two colliding black holes finally reaches the shores of Earth, it has diminished significantly in size. LIGO, for example, is built to detect variations in distance created by a gravitational wave. If LIGO's detectors were 93 million miles long, the variation in distance caused by a gravitational wave would still be less than the width of a human hair.
PTAs also make extremely sensitive measurements, but instead of distance they measure variations in the time between pulsar pulses. In the best-timed pulsars, scientists can measure variations down to about 100 billionths of a second.
Very few pulsars have this hairline level of accuracy, so PTAs combine observations from multiple pulsars on the sky to amplify faint gravitational-wave signals. Right now, scientists know of 2,500 pulsars in total, McLaughlin said. Only about 240 of those are the very fast pulsars, and even fewer of those turn out to be useful for gravitational-wave searches. Nanograv currently has 50 pulsars it uses for gravitational-wave searches and timing studies, 12 of which have a timing precision of less than 200 billionths of a second.
The future is bright
In her talk before the American Physical Society, McLaughlin said she and her colleagues in Nanograv think a gravitational wave detection by a PTA "could very easily be within the next five years."
There is debate in the PTA community about that estimate. George Hobbs is a member of the Parkes Pulsar Timing Array (PPTA), a group that keeps time on a cluster of pulsars using the Parkes Radio Telescope in New South Wales, Australia. (The PPTA and Nanograv are both part of the International Pulsar Timing Array group, along with the European Pulsar Timing Array.)
In September 2015, Hobbs and other members of PPTA published a paper in the journal Science in which they concluded that the gravitational-wave background must be so faint that it affects the timing of the pulsars by one billionth of a second or less.
That conclusion affects models that try to predict how gravitational waves are produced in the universe. If the gravitational waves are only affecting pulsars by one billionth of a second, then any models that predict a stronger signal have to be thrown out, and new ones have to be made.
According to the paper by the PPTA group, those new models show that the gravitational-wave background may be significantly fainter than PTAs are currently equipped to detect. Hobbs, who is also a research scientist in astronomy and space science at the Commonwealth Scientific and Industrial Research Organization (CSIRO) in Australia, told Space.com that for that reason, he and some of his colleagues feel that PTAs are unlikely to make a prediction in less than five years.
"So, we now think that the actual gravitational-wave amplitude is much lower than we had originally expected." Hobbs said. "If our pulsars remain stable then we'll get there in the end, but it will take much longer."
He estimates it will likely be at least 5 to 10 years before PTAs make a gravitational wave detection.
Members of Nanograv (including McLaughlin) published a response to the PPTA paper, arguing against part of the paper's premise; they also published two subsequent papers that suggest an earlier detection is possible . The variables that feed into those predictions still have huge uncertainties, McLaughlin said. For example, scientists don't know how many new pulsars the PTA's might be able to add to their arrays in the next few years, and they don't know the strength of the gravitational wave background signal.
But talking to Hobbs and McLaughlin, their broad predictions for when a PTA will make a gravitational wave detection are virtually the same: 5 to 10 years. The difference in opinion arises when probing that timeline at a more precise level: McLaughlin thinks a detection in 5 years is highly probable, while Hobbs talks about the situation as if a detection in 5 years is possible but highly unlikely, and it seems as though he wouldn't be surprised if it took longer than 1 years to make a detection.
Hobbs said his concerns about the time until a detection are also influenced by the fact that two radio telescopes currently used to survey the sky and search for new pulsars, Parkes and Aricebo. along with the Green Bank Telescope, which is used by Nanograv, "are under threat of closure."
If there is disagreement over how many well-timed pulsars will be discovered in the next five years, there is also heightened anticipation about the number of new pulsars that will be discovered in the next few decades. There are new radio telescopes currently under construction or on their way to operation, including the Five hundred meter Aperture Spherical Telescope (FAST) in China. Reports from inside the country say construction has finished on FAST, making it the largest radio telescope ever built. It is scheduled to come online in two to three years, and is designed to have twice the sensitivity of the Aricebo telescope. The upcoming Square Kilometer Array (SKA) radio telescope could be even more sensitive than FAST, although construction is not scheduled to begin on SKA until 2018. All of those telescopes introduce the possibility of new pulsar discoveries, some of which might be precise enough for scientists to use — and thus offer an opportunity to add to PTAs' precision and discovery power.
With the prospect of a gravitational-wave detection potentially on the horizon (or just beyond it) McLaughlin said another concern in the community is how to handle a detection.
"We've been seeing all the steps that the LIGO team has taken to show that these detections, in particular the first one, of course, are real," McLaughlin said. "So it's making us think very carefully [about] when we get to that point, and we have this big announcement to make, what kind of things are the community going to want to see to be convinced that our detection is also a real detection."
Hopefully, the community won't have to wait long to solve that particular problem.
Follow Calla Cofield @callacofield. Follow us @Spacedotcom, Facebook and Google+. Original article on Space.com.
Join our Space Forums to keep talking space on the latest missions, night sky and more! And if you have a news tip, correction or comment, let us know at: community@space.com.
Calla Cofield joined Space.com's crew in October 2014. She enjoys writing about black holes, exploding stars, ripples in space-time, science in comic books, and all the mysteries of the cosmos. Prior to joining Space.com Calla worked as a freelance writer, with her work appearing in APS News, Symmetry magazine, Scientific American, Nature News, Physics World, and others. From 2010 to 2014 she was a producer for The Physics Central Podcast. Previously, Calla worked at the American Museum of Natural History in New York City (hands down the best office building ever) and SLAC National Accelerator Laboratory in California. Calla studied physics at the University of Massachusetts, Amherst and is originally from Sandy, Utah. In 2018, Calla left Space.com to join NASA's Jet Propulsion Laboratory media team where she oversees astronomy, physics, exoplanets and the Cold Atom Lab mission. She has been underground at three of the largest particle accelerators in the world and would really like to know what the heck dark matter is. Contact Calla via: E-Mail – Twitter