First Solid Sign that Matter Doesn't Behave Like Antimatter
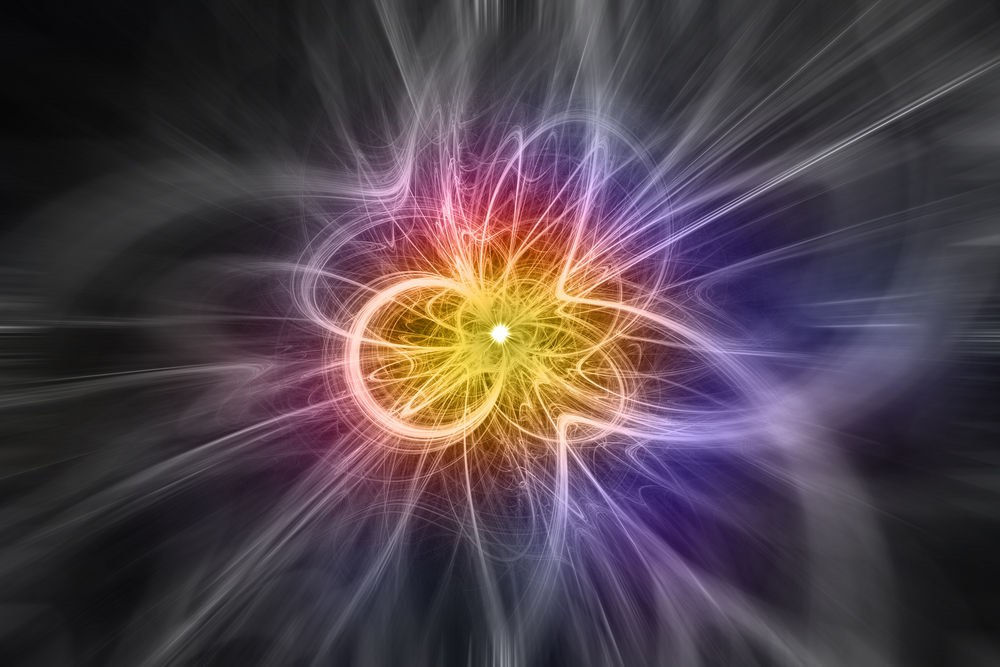
One of the biggest mysteries in physics is why there's matter in the universe at all. This week, a group of physicists at the world's largest atom smasher, the Large Hadron Collider, might be closer to an answer: They found that particles in the same family as the protons and neutrons that make up familiar objects behave in a slightly different way from their antimatter counterparts.
While matter and antimatter have all of the same properties, antimatter particles carry charges that are the opposite of those in matter. In a block of iron, for example, the protons are positively charged and the electrons are negatively charged. A block of antimatter iron would have negatively charged antiprotons and positively charged antielectrons (known as positrons). If matter and antimatter come in contact, they annihilate each other and turn into photons (or occasionally, a few lightweight particles such as neutrinos). Other than that, a piece of matter and antimatter should behave in the same way, and even look the same — a phenomenon called charge-parity (CP) symmetry. [The 18 Biggest Unsolved Mysteries in Physics]
Besides the identical behavior, CP symmetry also implies that the amount of matter and antimatter that was formed at the Big Bang, some 13.7 billion years ago, should have been equal. Clearly it was not, because if that were the case, then all the matter and antimatter in the universe would have been annihilated at the start, and even humans wouldn't be here.
But if there were a violation to this symmetry — meaning some bit of antimatter were to behave in a way that was different from its matter counterpart — perhaps that difference could explain why matter exists today.
To look for this violation, physicists at the Large Hadron Collider, a 17-mille-long (27 kilometers) ring beneath Switzerland and France, observed a particle called a lambda-b baryon. Baryons include the class of particles that make up ordinary matter; protons and neutrons are baryons. Baryons are made of quarks, and antimatter baryons are made of antiquarks. Both quarks and antiquarks come in six "flavors": up, down, top, bottom (or beauty), strange and charm, as scientists call the different varieties. A lambda-b is made of one up, one down and one bottom quark. (A proton is made of two up and one down, while a neutron consists of two down and one up quark.)
If the lambda and its antimatter sibling show CP symmetry, then they would be expected to decay in the same way. Instead, the team found that the lambda-b and antilambda-b particles decayed differently. Lambdas decay in two ways: into a proton and two charged particles called pi mesons (or pions), or into a proton and two K mesons (or kaons). When particles decay, they throw off their daughter particles at a certain set of angles. The matter and antimatter lambdas did that, but the angles were different. [7 Strange Facts About Quarks]
This is not the first time matter and antimatter have behaved differently. In the 1960s, scientists studied kaons themselves, which also decayed in a way that was different from their antimatter counterparts. B mesons — which consist of a bottom quark and an up, down, strange or charm quark — have also shown similar "violating" behavior.
Get the Space.com Newsletter
Breaking space news, the latest updates on rocket launches, skywatching events and more!
Mesons, though, are not quite like baryons. Mesons are pairs of quarks and antiquarks. Baryons are made of ordinary quarks only, and antibaryons are made of antiquarks only. Discrepancies between baryon and antibaryon decays had never been observed before.
"Now we have something for baryons," Marcin Kucharczyk, an associate professor at the Institute of Nuclear Physics of the Polish Academy of Sciences, which collaborated on the LHC experiment, told Live Science. "When you'd observed mesons, it was not obvious that for baryons it was the same."
While tantalizing, the results were not quite solid enough to count as a discovery. For physicists, the measure of statistical significance, which is a way of checking whether one's data could happen by chance, is 5 sigma. Sigma refers to standard deviations, and a 5 means that there is only a 1 in 3.5 million chance that the results would occur by chance. This experiment got to 3.3 sigma — good, but not quite there yet. (That is, 3.3 sigma means that there's about a 1 in 4,200 chance that the observation would have occurred randomly, or about a 99-percent confidence level.)
The findings are not a complete answer to the mystery of why matter dominates the universe, Kucharczyk said.
"It cannot explain the asymmetry fully," he said. "In the future, we will have more statistics, and maybe for other baryons."
The findings are detailed in the Jan. 30 issue of the journal Nature Physics.
Original article on Live Science.
Join our Space Forums to keep talking space on the latest missions, night sky and more! And if you have a news tip, correction or comment, let us know at: community@space.com.
Jesse Emspak is a freelance journalist who has contributed to several publications, including Space.com, Scientific American, New Scientist, Smithsonian.com and Undark. He focuses on physics and cool technologies but has been known to write about the odder stories of human health and science as it relates to culture. Jesse has a Master of Arts from the University of California, Berkeley School of Journalism, and a Bachelor of Arts from the University of Rochester. Jesse spent years covering finance and cut his teeth at local newspapers, working local politics and police beats. Jesse likes to stay active and holds a fourth degree black belt in Karate, which just means he now knows how much he has to learn and the importance of good teaching.