Long-Ago Smashups Could Explain Earth's Weird Composition
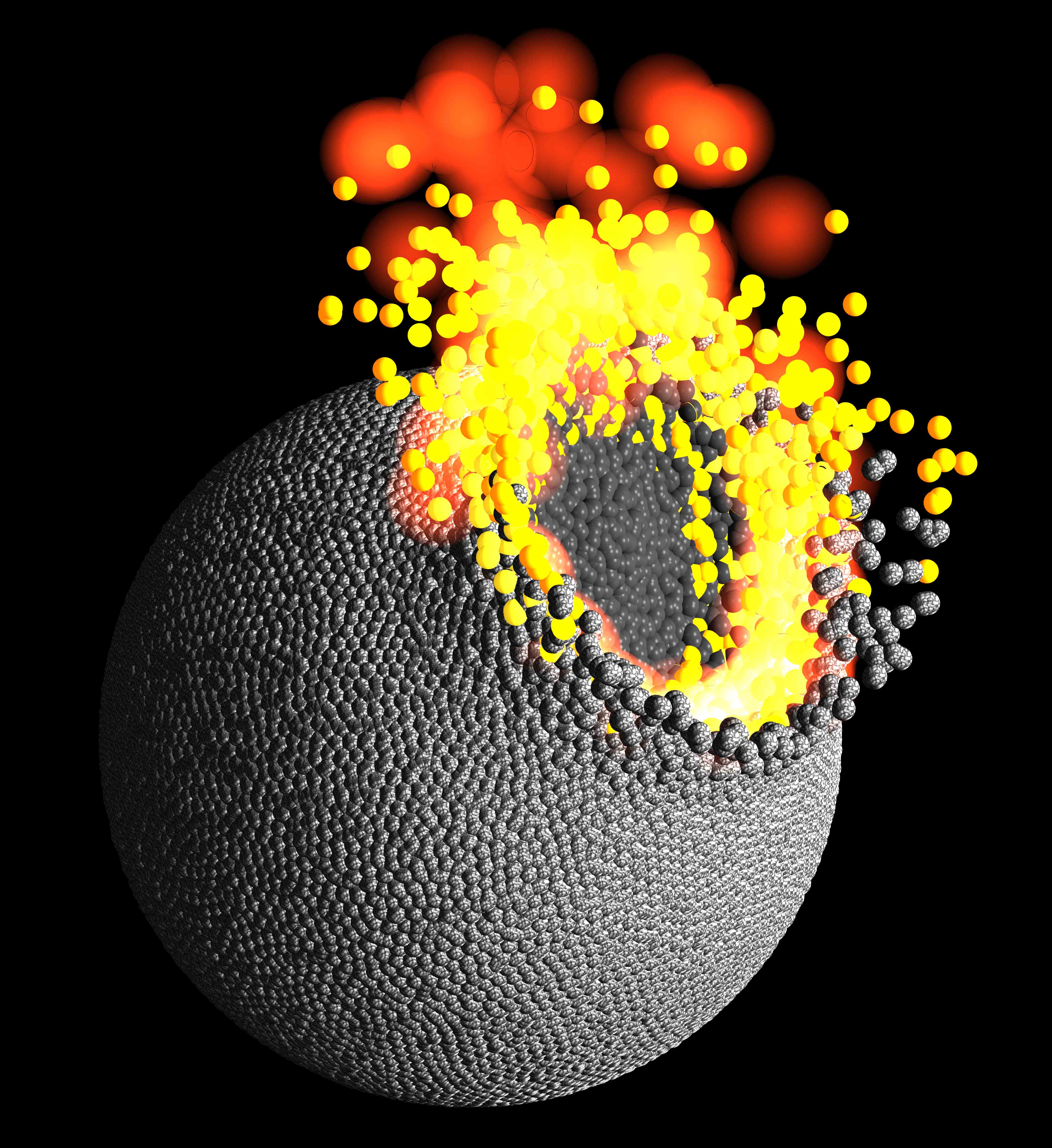
Earth and the other rocky planets have a composition that's different from the building blocks that formed them long ago — and scientists may now know why.
Collisions among these coalescing building blocks, known as planetesimals, generated enough heat to vaporize large amounts of rock, much of which escaped into space, suggest two new studies published today (Sept. 27) in the journal Nature.
"This vapor mass loss, in turn, drastically changes the composition of planets, which, in fact, explains Earth’s distinct composition," said Remco Hin, of the University of Bristol in England, who led one of the two new studies. [Photo Timeline: How the Earth Formed]
That composition is marked by a depletion of volatile rock-forming elements — the ones with a lower boiling point, which are more easily lost — compared to planetesimals. (Scientists have a good idea of planetesimal composition based on their analyses of certain meteorites known as chondrites, which are thought to be remnants of these ancient bodies.)
Hin and his colleagues measured the abundances of two isotopes of magnesium — Mg-24 and Mg-25 — in a variety of chondrites, Earth rocks and Mars meteorites. Isotopes are versions of an element that differ in the number of neutrons in their atomic nuclei; Mg-25 has one more neutron than Mg-24 does.
The researchers found the Earth and Mars rocks to be slightly depleted in Mg-24 compared to the chondrites. The implication is that planet-building collisions preferentially drove off the lighter Mg-24, while the heavier Mg-25 was better able to stick around.
The second study paints a similar picture. Ashley Norris and Bernard Wood, both of Oxford University in England, melted basaltic rock from Iceland in a furnace at 2,370 degrees Fahrenheit (1,300 degrees Celsius), then analyzed the volatile rock-forming elements that boiled away.
Get the Space.com Newsletter
Breaking space news, the latest updates on rocket launches, skywatching events and more!
"We find that the pattern of volatile element depletion in the silicate Earth is consistent with partial melting and vaporization, rather than with simple accretion of a volatile-rich chondrite-like body," Norris and Wood wrote in their study.
The second part of the above statement refers to a competing hypothesis — that our planet's composition can be explained by the long-ago collision of a roughly Mars-size body with a volatile-free proto-Earth, along with the migration of some elements to Earth's core. If this basic scenario sounds familiar, it should: Astronomers think such a giant impact led to the formation of Earth's moon about 4.5 billion years ago.
Indeed, Norris and Wood suggest that the moon-forming impact could have played a role in baking volatiles out of the early Earth. But they identify collisions among planetesimals as the likely dominant driver — a position with which Hin agrees.
Extremely high temperatures would have been required to drive volatiles away from the early Earth, Hin pointed out; the planet's powerful gravity would have held on to material much more strongly than that of planetesimals. And most (but not all) researchers don't think the moon-forming impact would have generated high enough temperatures to cause significant vapor loss, he said.
"So, it may be possible that some of the mass loss resulted from the Giant Impact, but it’s much less likely than from planetesimals, as we argued," Hin told Space.com via email. "Importantly, we see the same tell-tale Mg isotope feature of vapor loss in meteorite samples of the asteroid Vesta as we do in Earth. This suggests that the principle phase of vapor loss occurs in bodies this size or smaller."
The two new studies don't answer every question about the composition and formation of Earth, of course; many mysteries remain.
"Mars and Earth, for example, have different silicon isotope ratios that are not easily explained by the authors’ models," Edward Young, of the Department of Earth, Planetary, and Space Sciences at UCLA, wrote in an accompanying "News and Views" commentary in the same issue of Nature.
"Moreover, the isotopic effects of elements such as silicon and iron dissolving in the cores of planetary bodies must be accounted for," added Young, who was not a part of either study team. "Distinguishing the effects of core formation from those of vapor loss on isotopic compositions will take further study."
Follow Mike Wall on Twitter @michaeldwall and Google+. Follow us @Spacedotcom, Facebook or Google+. Originally published on Space.com.
Join our Space Forums to keep talking space on the latest missions, night sky and more! And if you have a news tip, correction or comment, let us know at: community@space.com.
Michael Wall is a Senior Space Writer with Space.com and joined the team in 2010. He primarily covers exoplanets, spaceflight and military space, but has been known to dabble in the space art beat. His book about the search for alien life, "Out There," was published on Nov. 13, 2018. Before becoming a science writer, Michael worked as a herpetologist and wildlife biologist. He has a Ph.D. in evolutionary biology from the University of Sydney, Australia, a bachelor's degree from the University of Arizona, and a graduate certificate in science writing from the University of California, Santa Cruz. To find out what his latest project is, you can follow Michael on Twitter.