Weird Neutrinos Can't Quite Explain Matter's Huge Riddle Yet
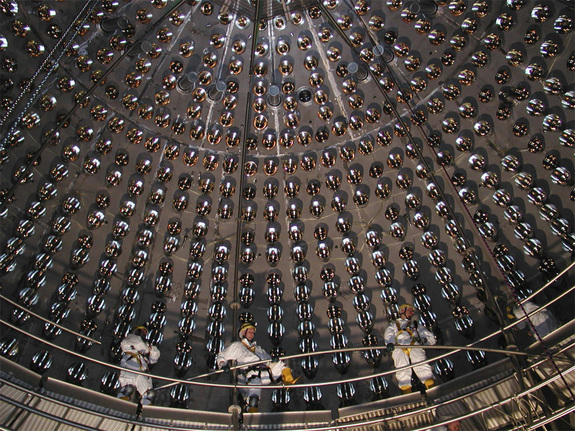
Deep below a mountain in Italy, in the coldest cubic meter of the known universe, scientists are hunting for evidence that ghostly particles called neutrinos act as their own antimatter partners. What these researchers find could explain the imbalance of matter and antimatter in the universe.
So far, they have come up empty-handed.
The latest results from the first two months of the CUORE (Cryogenic Underground Observatory for Rare Events) experiment in Gran Sasso, Italy, show no hint of a process proving neutrinos, which are generated by cosmic radiation, are their own antimatter partners. This means if the process does occur, it happens so rarely that it takes place roughly once every 10 septillion (10^25) years.
The ultimate goal of this experiment is to solve one of the universe's most enduring riddles, and one that suggests we shouldn't even be here. That riddle exists because the theoretical Big Bang — in which a tiny singularity is said to have inflated over 13.8 billion or so years to form the universe — should have resulted in a universe with 50 percent matter and 50 percent antimatter. [Big Bang to Civilization 10 Amazing Origin Events]
When matter and antimatter meet up, they annihilate and render each other nonexistent.
But that's not what we see today. Instead, our universe is mostly matter, and scientists are struggling to discover what happened to all the antimatter.
That's where neutrinos come in.
Get the Space.com Newsletter
Breaking space news, the latest updates on rocket launches, skywatching events and more!
What are neutrinos?
Most elementary particles have an odd antimatter counterpart, called an antiparticle, which has the same mass as its normal-matter partner but the opposite charge. But neutrinos are a little odd on their own, in that they hardly have any mass, and they are chargeless. So, physicists have surmised, they could be their own antiparticles.
When a particle acts as its own antiparticle, it's called a Majorana particle.
"The theories that we presently have simply don't tell us whether or not neutrinos are of that Majorana type. And it's a very interesting thing to look for, because we already know that we are missing something about the neutrinos," theoretical physicist Sabine Hossenfelder, a fellow at the Frankfurt Institute for Advanced Studies in Germany, told Live Science. Hossenfelder, who is not part of CUORE, is referring to the bizarre unexplained features of neutrinos.
If neutrinos are Majoranas, then they'd be able to transition between matter and antimatter. If most of the neutrinos morphed into ordinary matter at the universe's inception, the researchers said, this could explain why matter outweighs antimatter today — and why we exist.
The CUORE experiment
Studying neutrinos in a typical laboratory is difficult, because they rarely interact with other matter and are extremely hard to detect — billions pass through you undetected every minute. It's also hard to tell them apart from other sources of radiation. That's why physicists needed to go underground — nearly a mile (1.6 kilometers) below the Earth's surface — where a giant steel sphere encases a neutrino detector run by the Italian National Institute for Nuclear Physics' Gran Sasso National Laboratory. [5 Mysterious Particles That May Lurk Beneath Earth's Surface]
This laboratory is the home of the CUORE experiment, which is looking for evidence of a process called neutrinoless double-beta decay — another way of saying neutrinos act as their own antiparticles. In a normal double-beta decay process, a nucleus decays and emits two electrons and two antineutrinos. However, neutrinoless double-beta decay wouldn't emit any antineutrinos, because these antineutrinos could serve as their own antiparticles and would annihilate each other.
In their attempt to "see" this process, the physicists watched for the energy emitted (in the form of heat) during the radioactive decay of an isotope of tellurium. If neutrinoless double-beta decay occurred, there would be a peak at a certain energy level.
As the radioactive tellurium atoms decay, these detectors look for that energy peak.
The CUORE collaboration — a team of 200 scientists, engineers and technicians — released their first results. Their new paper, which was published March 26 in the journal Physical Review Letters,reveals that the experiment has not shown any neutrinoless double-beta decay after two months. However, they said they plan to continue the experiment for another five years to gather more data — the amount of time necessary to definitely rule out (or find) neutrinoless double-beta decay.
"The observation that neutrinos are their own antiparticles would be a significant discovery and require us to rewrite the commonly accepted Standard Model of particle physics. It would tell us that there is a new and different mechanism for matter to have mass," study researcher Karsten Heeger, a professor at Yale University, told Live Science.
And even if CUORE cannot definitively show that the neutrino is its own antiparticle, the technology used in the study may have other uses, said Lindley Winslow, an assistant professor of physics at the Massachusetts Institute of Technology and part of the CUORE team.
"The technology that cools CUORE down to 10 mK is the same that is used to cool superconducting circuits for quantum computing. The next generation of quantum computers may live in a CUORE-style cryostat [a device that keeps the temperature extremely cold]. You could call us early adopters," Winslow told Live Science.
Originally published on Live Science.
Join our Space Forums to keep talking space on the latest missions, night sky and more! And if you have a news tip, correction or comment, let us know at: community@space.com.