Churning spacetime and destroyed stars help reveal how fast supermassive black holes spin
"Frame dragging is an effect present around all spinning black holes."
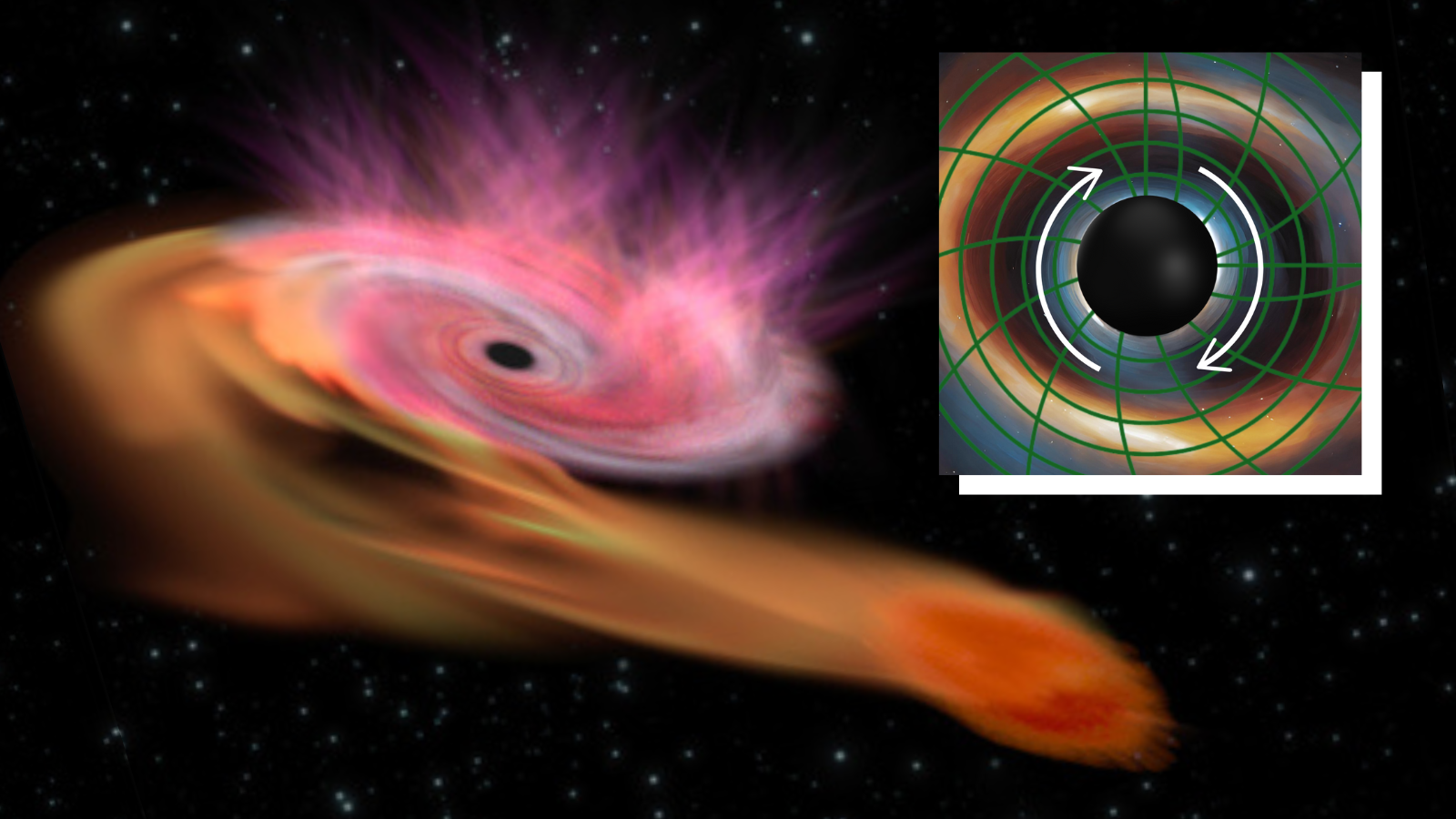
The "wobbling" remains of a star that suffered a grisly death at the maw of a supermassive black hole has helped reveal the speed at which its cosmic predator spins.
Supermassive black holes are believed to be born through successive mergers of smaller black holes, each of which brings with it angular momentum that speeds up the rotation of the black hole they birth. Consequently, measuring the spin of supermassive black holes can grant insight into their history — and new research offers a new way to make such inferences based on the effect spinning black holes have on the very fabric of space and time.
The doomed star at the heart of this research was ripped apart in brutal fashion by a supermassive black hole during a so-called tidal disruption event (TDE). These events are kicked off when a star ventures too close to the massive gravitational influence of a black hole. Once close enough, immense tidal forces are generated within the star, which squash it horizontally while stretching it vertically. That's called "spaghettification," and its a process that turns the star into a strand of stellar pasta — but, crucially, not all of it is gobbled by the destructive black hole.
Some of this material is blown away, while some of it wraps around the black hole, forming a flattened cloud called an accretion disk. Not only does this accretion disk gradually feed the central black hole, but the same tidal forces that shredded the star in the first place also cause massive friction forces that heat this platter of gas and dust, causing it to glow brightly.
Related: Scientists find black hole spaghettifying star remarkably close to Earth
Furthermore, when supermassive black holes spin, they drag along with them the very fabric of spacetime (a 4-dimensional unity of space and time). This so-called "Lense-Thirring" or "frame-dragging" effect means nothing stands still at the edge of a spinning supermassive black hole. The effect also causes a short-lived "wobble" in a newly formed black hole accretion disk.
Now, a team of researchers has discovered that the "wobble" of that accretion disk can be used to determine how fast the central black hole is spinning.
Get the Space.com Newsletter
Breaking space news, the latest updates on rocket launches, skywatching events and more!
"Frame dragging is an effect present around all spinning black holes," team leader Dheeraj "DJ" Pasham, a scientist at Massachusetts Institute of Technology (MIT), told Space.com. "So, if the disrupting black hole is spinning, then the flow of the stellar debris onto the black hole following a TDE is subject to this effect."
Holy hot X-ray stellar pasta!
To investigate TDEs and frame-dragging, the team spent five years searching for bright and relatively close examples of black-hole-induced stellar murders that could be quickly followed up on. The goal was to spot signs of accretion disk precession caused by the Lense-Thirring effect.
In February of 2020, this search reached fruition. The team managed to detect AT2020ocn, a bright flash of light coming from a galaxy located around billion light years away. AT2020ocn was initially spotted in optical light wavelengths by the Zwicky Transient Facility, with this visible-light data indicating the emission originated from a TDE involving a supermassive black hole with a mass between 1 million and 10 million times that of the sun.
"Because of the Lense-Thirring effect, the X-ray emission coming from the newly formed, hot accretion disk precesses, or 'wobbles.' This manifests as X-ray modulations in the data," Pasham said. "However, after a while, when accretion power comes down, gravity forces the disk to align with the black hole, after which the wobbling and the X-ray modulations stop."
Pasham and colleagues suspected the TDE that launched AT2020ocn could be the ideal event around which to hunt Lense-Thirring precession — and because this type of wobble is only present early after an accretion disk is formed, they had to act quickly.
"The key was to have the right observations," Pasham said. "The only way you can do this is, as soon as a tidal disruption event goes off, you need to get a telescope to look at this object continuously, for a very long time, so you can probe all kinds of timescales, from minutes to months."
That's where NASA's Neutron Star Interior Composition ExploreR (NICER) comes in: an X-ray telescope situated on the International Space Station (ISS) that measures X-ray radiation around black holes and other ultradense, compact massive objects like neutron stars. The team found NICER was not only able to catch the TDE, but the ISS-mounted X-ray telescope was also able to continuously monitor the event as it developed over several months.
"We discovered that the X-ray brightness and the temperature of the region emitting X-rays following a TDE to be modulating on a timescale of 15 days," Pasham said. "This recurring 15-day X-ray signal went away after three months."
The team's findings also delivered a surprise.
Estimates of the black hole’s mass and the mass of the disrupted star revealed that the black hole wasn't spinning as fast as expected. "It was slightly surprising that the black hole is not spinning that fast — only less than 25% the speed of light," Pasham said.
Pasham thinks that, thanks to the upcoming Vera C. Rubin Observatory, currently under construction in northern Chile and set to conduct a 10-year survey of the universe called the Legacy Survey of Space and Time (LSST), the future is bright for TDE-hunting.
"Rubin is expected to detect thousands of TDEs over the next decade. If we can measure Lense-Thirring precession in even a small fraction of them, we will be able to say something about the spin distribution of supermassive black holes, which is coupled with how they evolved over the age of the universe," Pasham concluded. "Our team has a couple of observing proposals lined up to follow up on future TDEs. We'll be investigating frame-dragging around other TDE black holes for sure!"
The team's research was published on Wednesday (May 22) in the journal Nature.
Join our Space Forums to keep talking space on the latest missions, night sky and more! And if you have a news tip, correction or comment, let us know at: community@space.com.
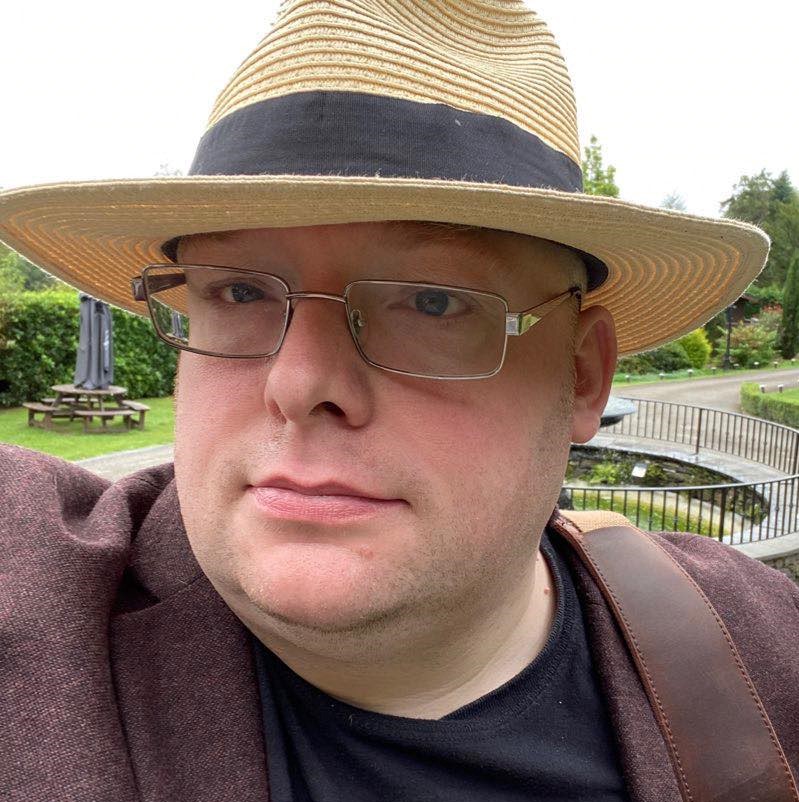
Robert Lea is a science journalist in the U.K. whose articles have been published in Physics World, New Scientist, Astronomy Magazine, All About Space, Newsweek and ZME Science. He also writes about science communication for Elsevier and the European Journal of Physics. Rob holds a bachelor of science degree in physics and astronomy from the U.K.’s Open University. Follow him on Twitter @sciencef1rst.
NASA spacecraft spots monster black hole bursting with X-rays 'releasing a hundred times more energy than we have seen elsewhere'
Could we use black holes to power future human civilizations? 'There is no limitation to extracting the enormous energy from a rotating black hole'