How did the universe's elements form?
The journey of the elements starts in the earliest moments of the Big Bang, when our universe was only a few seconds to a few minutes old.
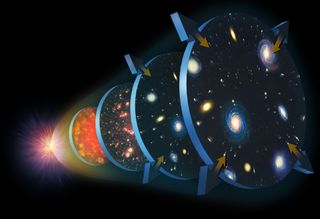
We all know the universe contains a vast array of elements, ranging from light gases, such as helium, to heavy metals, like lead. But where did all of the elements come from?
The journey of the elements starts in the earliest moments of the Big Bang, when our universe was only a few seconds to a few minutes old. At that time, the entire cosmos was crammed into a volume millions of times smaller than it is today. Due to the incredibly high densities, the average temperature of all the material in the universe was well over a billion degrees, which is more than hot enough for nuclear reactions to take place. In fact, it was so hot that even protons and neutrons could not exist as stable entities. Instead, the universe was just a sea of more fundamental particles, called quarks and gluons, seething in a raw plasma state.
But the universe would not stay that way for long. It was expanding, which means it was also cooling. Eventually, the quarks could bind together to form the first protons and neutrons without instantly getting demolished. Protons are ever so slightly lighter than neutrons, which gave them an edge in this initial phase of particle production. Once the universe was a few minutes old, it was far too cold to create new protons and neutrons. So those first heavy particles were the only ones the universe was ever going to make (outside of future rare high-energy interactions).
Related: The history of the universe: Big Bang to now in 10 easy steps
By the time the heavy particles finally froze out, there were roughly six protons for every neutron. Neutrons by themselves aren't stable; they decay with a half-life of around 880 seconds. Immediately, some of the neutrons began to decay away, while the remainder started binding with protons to form the first atomic nuclei. Of all the light elements, helium-4, which consists of two protons and two neutrons, has the largest binding energy, which means it's the easiest to form and the hardest to break apart. So almost all of those neutrons went into the production of helium-4.
From calculations like this, cosmologists can predict that the universe started out with a mixture of roughly 75% hydrogen (which is just a bare proton), 25% helium and a small scattering of lithium — which is exactly what astronomers observe.
Stellar nucleosynthesis
The next stage in the appearance of the elements had to wait for the first generation of stars, which didn't start shining until hundreds of millions of years after the Big Bang. Stars power themselves through nuclear fusion, transforming hydrogen into helium. This process leaves a tiny bit of energy left over. But stars have so much hydrogen available that they can burn for billions, or sometimes trillions, of years.
Get the Space.com Newsletter
Breaking space news, the latest updates on rocket launches, skywatching events and more!
Near the ends of their lives, stars like the sun switch to fusing helium instead, turning it into carbon and oxygen before they die as planetary nebulae. This is why carbon and oxygen are so abundant in the universe; after hydrogen and helium, they are the most commonly produced elements. In fact, oxygen is the most common element on Earth, although most of it is bound up with silicates to form the ground beneath your feet.
More massive stars — those with at least eight times the mass of the sun — fuse even heavier elements in their cores. Especially in their final weeks, days and even hours, the most massive stars in the universe create nitrogen, neon, silicon, sulfur, magnesium, nickel, chromium and iron.
Aftermath nucleosynthesis
That's the end of the line for the formation of elements within stars. Their intense energies are perfectly capable of producing heavier elements, but fusing anything above iron saps energy, rather than producing it, so those heavier elements appear only rarely in the cores of massive stars.
Instead, the rest of the elements in the periodic table are produced when stars die, which they do through a variety of fascinating, complicated and spectacular means. Smaller stars slowly turn themselves inside out, spewing their guts all across their stellar systems. Larger stars explode in violent cataclysms known as supernovas. Both kinds of deaths leave remnants. In the case of small stars, they leave white dwarfs, which are made almost entirely of carbon and oxygen. Larger stars leave behind incredibly dense spheres of neutrons known as neutron stars.
Gas from a companion star can fall onto a white dwarf, causing it to trigger its own kind of supernova blast. Neutron stars can collide with each other, releasing an enormous amount of energy in an event known as a kilonova.
No matter what, all of these processes involve a lot of radiation, a lot of energy and a lot of particles flying around at high speed — in other words, the perfect soup for fashioning new elements. It's through these calamities that the rest of the periodic table came into being.
It's also through these energetic events that these elements spread beyond the bounds of their home stars and out into the interstellar mix. There, those elements join new gas clouds, which eventually coalesce to form new generations of stars that continue the process of elemental recycling and regeneration, slowly enriching the universe.
Join our Space Forums to keep talking space on the latest missions, night sky and more! And if you have a news tip, correction or comment, let us know at: community@space.com.
Paul M. Sutter is an astrophysicist at SUNY Stony Brook and the Flatiron Institute in New York City. Paul received his PhD in Physics from the University of Illinois at Urbana-Champaign in 2011, and spent three years at the Paris Institute of Astrophysics, followed by a research fellowship in Trieste, Italy, His research focuses on many diverse topics, from the emptiest regions of the universe to the earliest moments of the Big Bang to the hunt for the first stars. As an "Agent to the Stars," Paul has passionately engaged the public in science outreach for several years. He is the host of the popular "Ask a Spaceman!" podcast, author of "Your Place in the Universe" and "How to Die in Space" and he frequently appears on TV — including on The Weather Channel, for which he serves as Official Space Specialist.
-
rod "Instead, the universe was just a sea of more fundamental particles, called quarks and gluons, seething in a raw plasma state."Reply
My observation. I did not see how even earlier times in cosmology created this *raw plasma state*. Consider the inflaton, how did the inflaton create quarks and gluons? -
billslugg I don't think the inflation created anything, it is just a debate over expansion coefficient. Here is what happened, they looked at the size of the quantum fluctuations at the first instant in time of the BB and saw a certain size of fluctuation in a certain size of Universe.Reply
Then they looked at the size of the Universe today as compared to the size of today's fluctuations and they said: "This does not add up!". The fluctuations today are way, way smaller that what would have predicted based on how much smaller the variations become as things stretch out.
THUS: The Universe must have done a lot more expansion than we thought thus it must have been very much smaller at the start than we thought, thus there must have been a period of great expnsion we shall call "Inflation".
That's as I understand it. -
Helio Yes, IIRC, quarks came after Inflation where temperatures were still too high for quarks. Inflation is estimated at ending at 10E-35 sec., though hard science can only address time to 10E-12 sec.Reply
It was Guth, primarily, who stepped in to address two new challenges BBT presented as time was extrapolated backwards. These were the “horizon“ and ”flatness” problems.
BBT is baseed on nearly pure homogeneity. But quantum science near t=o requires a far less degree of uniformity. But the CMBR is smooth to 1part in 100,000, too smooth for what we should see.
Also, the universe is almost perfectly in balance where gravity is equally offset by the expansion force,
Inflation happens to solve both of those problems, apparently.
But, when something that is crazy hard to test gets tossed into a theory to fix problems, it can be deemed as ad hoc. Alternatives, no doubt, to Inflation are welcome.
What’s important, IMO, is how much we’ve discovered that has revealed just how fine-tuned our universe is, as if by design, allowing stars to form. elements to be manufactured, planets to form, and life to come forth. -
Atlan0001 Ignoring the microcosmic Planck (the macrocosmic Big Bang) singular level 'Horizon' of the cosmic aren't we.Reply
In Chaos Theory, in fractal zoom universe structure (geometric progression structure (square matrix)), the dimensional horizon of seamlessly smooth simplicity alternates in onion skin layering with the dimensional horizon of chunky grained complexity, a single constant existing all at once of set and spontaneous reset to infinity. A Schrodinger function of one and the other / one or the other.
Hawking described in 'A Brief History of Time' a life zone of universe where life is always -- forever -- in migration to stay in the life zone ("Grand Central Station"). A sort of forever moving hyperspatial constant of (Tolkien-like) 'Middle Earth' 'middle universe' (chunky complexity level) bracketed (smooth simplicity level). The twain is twain but blends in 'Horizon' as we do in Earth's horizon but with a big difference.
Related . . . if one can see it:
https://www.thestreet.com/technology/neil-degrasse-tyson-says-one-wild-sci-fi-discovery-would-completely-change-the-global-economy -
Atlan0001 In the illustration of the wormhole in the URL pointed to in my post #5, keep the sheet going, keep the folds going, and keep the piping going through of the wormhole, to infinity. The wormhole, in the case I presented above, encompasses the entire 'middle universe' of Hawking's description of the migrating life zone and migration of life, and my own description of keeping constant to the dimensional horizon fractal zoom universe structure of chunky grained complexity . . . receding horizon to receding horizon, to receding horizon..., (middle universe to middle universe, to middle universe....). A sheet of endlessly (spontaneous) emergent beginning (seamlessly smooth simplicity) and a nice big wormhole middle universe (fractal zoom universe structure, set and reset) of endless life zone in an endless frontier of chunky grained complexity. Each stratum! a continuum of strata!Reply
Set and reset via hyper-wormhole, sort of like an endless frontier into itself of a 'Klein bottle'!