How heavy is the universe? Conflicting answers hint at new physics.
Standard model of cosmology may need a rewrite.
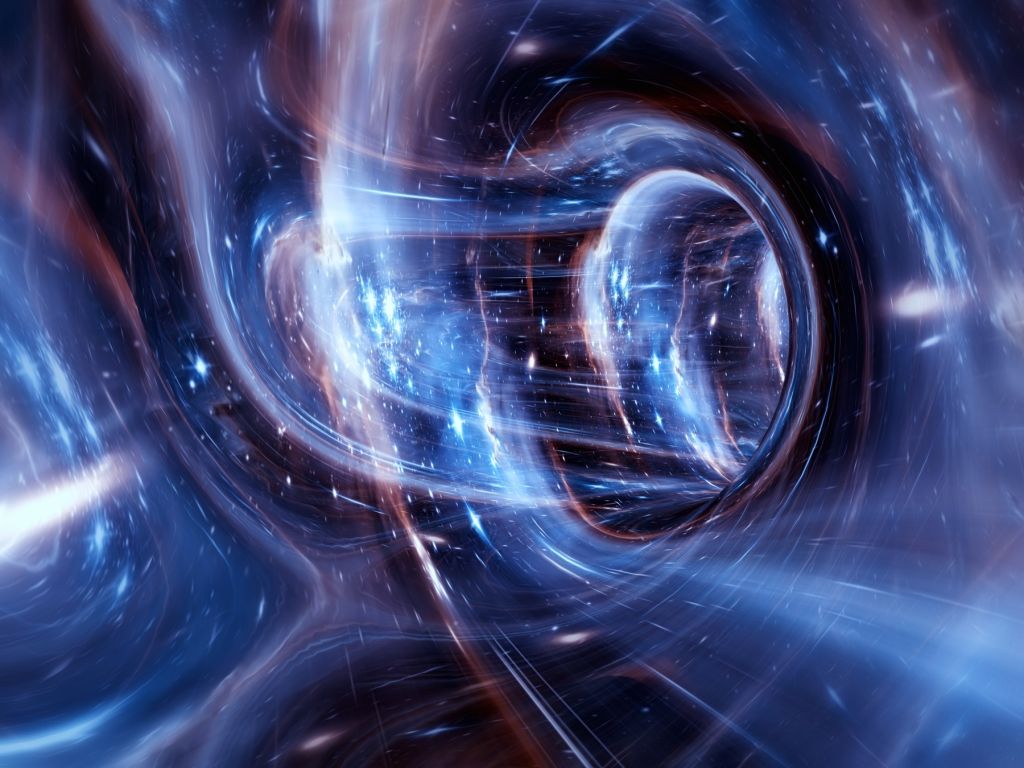
Two entirely different ways of "weighing" the cosmos are producing disparate results. If more precise measurements fail to resolve the discrepancy, physicists may have to revise the standard model of cosmology, our best description of the universe.
"If this really is a glimpse of the standard model breaking down, that would be potentially revolutionary," says astronomer Hendrik Hildebrandt of the Ruhr University Bochum in Germany.
Similar concerns over the correctness of the standard model have been raised over the past few years by two independent calculations of the so-called Hubble constant, or the rate at which the universe is expanding today. Those two measurements also disagreed, creating what has been called the Hubble tension.
The new discrepancy — called the sigma-eight tension — involves measuring the density of matter in the universe and the degree to which it is clumped up, as opposed to being uniformly distributed. The result is encapsulated in a parameter called sigma-eight. To calculate sigma-eight, Hildebrandt and his colleagues turned to an effect called weak gravitational lensing, in which the light from distant galaxies is bent ever so slightly toward our telescopes because of the gravitational pull from matter that lies between the galaxies and Earth.
The resulting distortion is so small that it barely changes the shape of an individual galaxy. But if you take an average of the shapes of tens of thousands of galaxies in a patch of sky, a signal of weak lensing pops out. Presuming that galaxies should be randomly oriented with respect to Earth, their average shape should be nearly circular—without weak lensing, that is. But thanks to the mild distortions from this effect, the average shape instead veers toward the elliptical.
The astronomers used this signal to estimate the amount and distribution of intervening matter (both normal and dark varieties) along the lines of sight to various galaxy-rich regions across a large patch of the sky. In other words, they managed to measure matter's cosmic density.
But doing so precisely requires one more piece of information: the distance to each individual galaxy being studied. Normally, astronomers calculate the distance to another galaxy by finding its spectroscopic redshift—the amount by which the galaxy's light is shifted toward the longer wavelengths of the red side of the spectrum. The greater the redshift, the farther away the object.
Get the Space.com Newsletter
Breaking space news, the latest updates on rocket launches, skywatching events and more!
Measuring individual spectroscopic redshifts, however, is extremely inefficient when dealing with millions of galaxies. So Hildebrandt's team turned to something called photometric redshift, which involves taking multiple images of the same patch of sky in different wavelengths, spanning the optical and near-infrared ranges. The researchers used those images to estimate the redshift of individual galaxies in each one. "They're not as good as the traditional spectroscopic redshift," Hildebrandt says. "But they're much more efficient in terms of telescope time."
For its entire analysis, the team used high-resolution images of hundreds of square degrees of the sky (the full moon is about half a degree across) in nine wavelength bands—four optical and five near-infrared. These observations of about 15 million galaxies were collected by the European Southern Observatory's Kilo-Degree Survey (KiDS) and VISTA Kilo-Degree Infrared Galaxy Survey (VIKING) using two small telescopes at the organization's Paranal Observatory in Chile.
The VIKING data bolstered the KiDS data set by providing multiple observations of the same region of the sky in near-infrared wavelengths. The greater the distances of a galaxy, the higher the speed at which it is receding from us. This causes more of a galaxy's light to be redshifted into the near-infrared range, so relying solely on optical observations is not enough. Infrared measurements capture a greater amount of the light from such galaxies, leading to better estimates of their photometric redshift.
To ensure that photometric redshifts are as accurate as possible, these observations were calibrated against spectroscopic redshift measurements of a few of the same galaxies made with the more massive eight-meter Very Large Telescope at Paranal and the 10-meter Keck telescopes on Mauna Kea in Hawaii.
Astrophysicist and Nobel laureate Adam Riess of Johns Hopkins University approves of the efforts of the KiDS researchers. "Their latest results use infrared data, which probably does a better job of tracing the mass of the lenses and getting reliable photometric redshifts," he says.
Using the combined data, covering about 350 square degrees of the sky, the astronomers estimated sigma-eight.The value they found conflicts with a sigma-eight figure calculated using the European Space Agency's Planck satellite's observations of the cosmic microwave background (CMB)—the earliest observable light in the universe, which was emitted about 380,000 years after the big bang. Planck mapped the variations in the temperature and polarization of the CMB from point to point in the sky. Cosmologists can employ the map to calculate the sigma-eight value for the early universe. Using the standard model of cosmology (which says that the cosmos is made of about 5 percent ordinary matter, 27 percent dark matter and 68 percent dark energy), they can then extrapolate across more than 13 billion years of cosmic evolution to estimate the present-day value for sigma-eight.
Herein lies the tension. Hildebrandt's weak-lensing study estimates sigma-eight to be about 0.74, whereas the Planck data provides a value of about 0.81. "There is about a 1 percent chance or so that this [tension] is a statistical fluctuation," Hildebrandt says. Statistical fluctuations are random noise in data that can mimic actual signals and can disappear with more data. "This is not something to completely lose sleep over."
Not yet anyway. It is also possible a systematic error lurks in the calculations of one or both of the teams. After the researchers identify any such errors, the discrepancy could go away.
Or it may not do so, which has been the case with the Hubble tension. As astronomical measurements have become more precise, the statistical significance of the Hubble tension has only grown, inflicting sleepless nights on more than a few anxious theorists."Something very similar might happen with our sigma-eight discrepancy," Hildebrandt says. "We don't know."
Riess, who leads one of the teams estimating the Hubble constant using measurements of supernovae in the nearby universe, likens the sigma-eight tension to a "little brother or sister of the Hubble tension." That discrepancy is now considered statistically significant, with less than a one-in-3.5-million chance of being a fluke. The sigma-eight tension, with its one-in-100 chance of being a statistical aberration, is where the Hubble tension was a few years ago. "So [it is] less significant but worth keeping an eye on for a possible connection," Riess says.
If the sigma-eight tension ascends to the same level of statistical relevance as the Hubble tension, the pressure to reevaluate the standard model of cosmology could become too enormous to ignore. At that point, cosmologists may be forced to invoke new physics to bring the Planck estimates in line with the direct measurements of the parameters of the present-day universe. "That will be the exciting alternative," Hildebrandt says.
Potential "new physics" fixes to the standard model could involve changing the amount and nature of dark energy or dark matter—or both—as well as tweaks to how they interact with each other and with normal matter, among other more exotic modifications. "Some theoretical solutions to tinker with the cosmological model to fix the Hubble constant tension make this [sigma-eight tension] worse. Some make it better," Riess says.
Hildebrandt agrees that there is no obvious solution in sight. "If there was a compelling model, maybe people would jump on that bandwagon," he says. "But at the moment, I don't think there is. It's really on us observers to improve the significance [of the sigma-eight tension] or disprove it."
This article was first published at ScientificAmerican.com. © ScientificAmerican.com. All rights reserved Follow Scientific American on Twitter @SciAm and @SciamBlogs. Visit ScientificAmerican.com for the latest in science, health and technology news.
OFFER: Save 45% on 'All About Space' 'How it Works' and 'All About History'!
For a limited time, you can take out a digital subscription to any of our best-selling science magazines for just $2.38 per month, or 45% off the standard price for the first three months.
Join our Space Forums to keep talking space on the latest missions, night sky and more! And if you have a news tip, correction or comment, let us know at: community@space.com.
-
rod "Potential "new physics" fixes to the standard model could involve changing the amount and nature of dark energy or dark matter—or both—as well as tweaks to how they interact with each other and with normal matter, among other more exotic modifications. "Some theoretical solutions to tinker with the cosmological model to fix the Hubble constant tension make this worse. Some make it better," Riess says. Hildebrandt agrees that there is no obvious solution in sight. "If there was a compelling model, maybe people would jump on that bandwagon," he says. "But at the moment, I don't think there is. It's really on us observers to improve the significance or disprove it."Reply
This is an interesting article. Consider that about 4% of the universe is readily observable and as one source i have said, "That leaves only about 4% of reality accessible to observation." The other 96% remains a struggle in the BB cosmology. -
LijeBaley It's all swag right now with almost no experimental evidence. At least Einstein made predictions and suggested experiments that would either confirm or disprove his theories. So far General Relativity has held up well to experimentation with each year brining actual data that confirms his theories, the latest being a photograph of a black hole's event horizon in M87. Quantum mechanics is still very much theory with little experimental evidence. Same goes for dark energy and dark matter. Calculations suggest it but who knows.Reply -
rod Good comment LijeBaley. I note this recent report searching for DM at the quantum level in experiments. No evidence of an influence of dark matter on the force between nuclei, "The universe mainly consists of a novel substance and an energy form that are not yet understood. This 'dark matter' and 'dark energy' are not directly visible to the naked eye or through telescopes...Dark matter interacts with normal matter via the gravitational force, which also determines the cosmic structures of normal, visible matter. It is not yet known whether dark matter also interacts with itself or with normal matter via the other three fundamental forces—the electromagnetic force, the weak and the strong nuclear force—or some additional force."Reply -
chemicalmicroscopist As a chemist I am not convinced that dark matter exists. Some other explanation is needed.Reply
It seems that the old gravimetric analytical methods would not so consistently agree with newer analytical methods involving mass spectrometry and coulometric methods or be so self consistent. Gravity will influence gravimetric methods but have no effect on MS or electro-chemical methods. If my sample or the earth contain dark matter it will throw off (cause inconsistencies) gravimetic measurements. OR is the physics community claiming there is no dark matter in our solar system? I have been watching for a reasonable explanation. Does anyone have one? -
rod chemicalmicroscopist said:As a chemist I am not convinced that dark matter exists. Some other explanation is needed.
It seems that the old gravimetric analytical methods would not so consistently agree with newer analytical methods involving mass spectrometry and coulometric methods or be so self consistent. Gravity will influence gravimetric methods but have no effect on MS or electro-chemical methods. If my sample or the earth contain dark matter it will throw off (cause inconsistencies) gravimetic measurements. OR is the physics community claiming there is no dark matter in our solar system? I have been watching for a reasonable explanation. Does anyone have one?
FYI. DM in our solar system has been discussed on some of the forum threads, one source cited a 2018 report claiming only an asteroid worth of DM would be in the solar system. I did some checking and found 2020 reports that contradicted such claims, ref - Measuring the local Dark Matter density in the laboratory, "Despite strong evidence for the existence of large amounts of dark matter (DM) in our Universe, there is no direct indication of its presence in our own solar system. All estimates of the local DM density, crucial for all direct DM searches, rely on extrapolating estimates on much larger scales. We demonstrate for the first time the possibility of measuring the local DM density with a direct detection experiment..."
ref - Gravitational Focusing of Low-Velocity Dark Matter on the Earth's Surface
Searches continue but so far, direct DM detection at Earth or in our solar system remains something yet to be confirmed it seems---Rod -
rod oops, looks like my first reference was not correctly cited, this should work. Measuring the local Dark Matter density in the laboratoryReply -
voidpotentialenergy Did they forget to measure the temp matter and temporary energy of quantum fluctuation particle creation/destruction.? Simple solution to the missing mass and dark energy.Reply
Large universe with many small temp mass and energy properties= large fleeting constant mass and energy. -
Torbjorn Larsson rod said:"Potential "new physics" fixes to the standard model could involve changing the amount and nature of dark energy or dark matter—or both—as well as tweaks to how they interact with each other and with normal matter, among other more exotic modifications. "Some theoretical solutions to tinker with the cosmological model to fix the Hubble constant tension make this worse. Some make it better," Riess says. Hildebrandt agrees that there is no obvious solution in sight. "If there was a compelling model, maybe people would jump on that bandwagon," he says. "But at the moment, I don't think there is. It's really on us observers to improve the significance or disprove it."
This is an interesting article. Consider that about 4% of the universe is readily observable and as one source i have said, "That leaves only about 4% of reality accessible to observation." The other 96% remains a struggle in the BB cosmology.
Well, it is not statistically significant enough result, and so opinions may differ - there are many such suggestions out there, and as the statistics suggest most go away with further observations.
On the contrary there is no "struggle" to predict dark matter or dark energy in LCDM, the observations fits very well and at low uncertainty. For a recent test, the new repetition of measuring intergalactic gas with 6 instead of 2 FBR radio sources pinned the 4 % normal matter perfectly.
I think you mean that we don't know much detail about dark energy or dark matter yet. But as the above example show, LCDM makes advances all the time. No need to "struggle". -
Torbjorn Larsson LijeBaley said:It's all swag right now with almost no experimental evidence. At least Einstein made predictions and suggested experiments that would either confirm or disprove his theories. So far General Relativity has held up well to experimentation with each year brining actual data that confirms his theories, the latest being a photograph of a black hole's event horizon in M87. Quantum mechanics is still very much theory with little experimental evidence. Same goes for dark energy and dark matter. Calculations suggest it but who knows.
Both quantum physics and LCDM has many and independent experimental evidences! The data that is observed and predicted by both are astronomical, see for example the LHC and the Planck surveys.
- LHC has analyzed 300 trillion (3\00d710^14) proton-proton collisions and store 25 petabyte observational data per year. *All* of it tests quantum physics well! https://en.wikipedia.org/wiki/Worldwide_LHC_Computing_Grid ]
- Planck has made a 4+ year, sky wide, multiple band analog survey. They too had to do sophisticated data processing to get it done https://arxiv.org/ftp/astro-ph/papers/0604/0604069.pdf ], and *all* of it tests LCDM well!
The amount of data generated and tested in astronomy is today ... well, astronomical.