40 quintillion stellar-mass black holes are lurking in the universe, new study finds
"Small" black holes are estimated to make up 1% of the universe's matter.
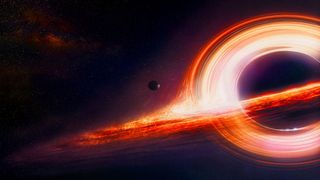
Scientists have estimated the number of "small" black holes in the universe. And no surprise: It's a lot.
This number might seem impossible to calculate; after all, spotting black holes is not exactly the simplest task. Because they're are as pitch-black as the space they lurk in, the light swallowing cosmic goliaths can be detected only under the most extraordinary circumstances — like when they're bending the light around them, snacking on the unfortunate gases and stars that stray too close, or spiraling toward enormous collisions that unleash gravitational waves.
But that hasn't stopped scientists from finding some ingenious ways to guess the number. Using a new method, outlined Jan. 12 in The Astrophysical Journal, a team of astrophysicists has produced a fresh estimate for the number of stellar-mass black holes — those with masses 5 to 10 times that of the sun — in the universe.
Related: The 12 strangest objects in the universe
And it's astonishing: 40,000,000,000,000,000,000, or 40 quintillion, stellar-mass black holes populate the observable universe, making up approximately 1% of all normal matter, according to the new estimate.
So how did the scientists arrive at that number? By tracking the evolution of stars in our universe they estimated how often the stars — either on their own, or paired into binary systems — would transform into black holes, said first author Alex Sicilia, an astrophysicist at the International School of Advanced Studies (SISSA) in Trieste, Italy.
"This is one of the first, and one of the most robust, ab initio [ground up] computation[s] of the stellar black hole mass function across cosmic history," Sicilia said in a statement.
Get the Space.com Newsletter
Breaking space news, the latest updates on rocket launches, skywatching events and more!
To make a black hole, you need to start with a large star — one with a mass roughly five to 10 times that of the sun. As big stars reach the end of their lives, they begin to fuse heavier and heavier elements, such as silicon or magnesium, inside their fiery cores. But once this fusion process begins forming iron, the star is on a path to violent self-destruction. Iron takes in more energy to fuse than it gives out, causing the star to lose its ability to push out against the immense gravitational forces generated by its enormous mass. It collapses in on itself, packing first its core, and later all the matter close to it, into a point of infinitesimal dimensions and infinite density — a singularity. The star becomes a black hole, and beyond a boundary called the event horizon, nothing — not even light — can escape its gravitational pull.
To arrive at their estimate, the astrophysicists modeled not just the lives, but the pre-lives of the universe's stars. Using known statistics of various galaxies, such as their sizes, the elements they contain, and the sizes of the gas clouds stars would form in, the team built a model of the universe that accurately reflected the different sizes of stars that would be made, and how often they would be created.
After pinning down the rate of formation for stars that could eventually transform into black holes, the researchers modeled the lives and deaths of those stars, using data such as their mass and a trait called metallicity — the abundance of elements heavier than hydrogen or helium — to find the percentage of candidate stars that would transform into black holes. By also looking at stars paired into binary systems, and by calculating the rate at which black holes can meet each other and merge, the researchers ensured that they weren’t double-counting any black holes in their survey. They also figured out how these mergers, alongside the snacking by black holes on nearby gas, would affect the size distribution of the black holes found across the universe.
With these calculations in hand, the researchers designed a model that tracked the population and size distribution of stellar-mass black holes over time to give them their eye-watering number. Then, by comparing the estimate with data taken from gravitational waves, or ripples in space-time, formed by black hole and binary star mergers, the researchers confirmed that their model was in good agreement with the data.
Astrophysicists hope to use the new estimate to investigate some perplexing questions that arise from observations of the very early universe — for instance, how the early universe became so quickly populated by supermassive black holes — often with masses millions, or even billions, of times greater than the stellar-mass holes the researchers examined in this study — so soon after the Big Bang.
Because these gigantic black holes came from the merging of smaller, stellar-mass black holes — or black hole 'seeds' — the researchers hope that a better understanding of how small black holes formed in the early universe could help them to unearth the origins of their supermassive cousins.
"Our work provides a robust theory for the generation of light seeds for supermassive black holes at high redshift [further back in time], and can constitute a starting point to investigate the origin of "heavy seeds", that we will pursue in a forthcoming paper," Lumen Boco, an astrophysicist at SISSA, said in the statement.
Originally published on Live Science.
Join our Space Forums to keep talking space on the latest missions, night sky and more! And if you have a news tip, correction or comment, let us know at: community@space.com.
Ben Turner is a U.K. based staff writer at Live Science. He covers physics and astronomy, among other topics like weird animals and climate change. He graduated from University College London with a degree in particle physics before training as a journalist. When he's not writing, Ben enjoys reading literature, playing the guitar and embarrassing himself with chess.