The Large Hadron Collider returns in the hunt for new physics
Firing particles at each other at 99.99% of the speed of light, the LHC is back in the race to discover new physics.
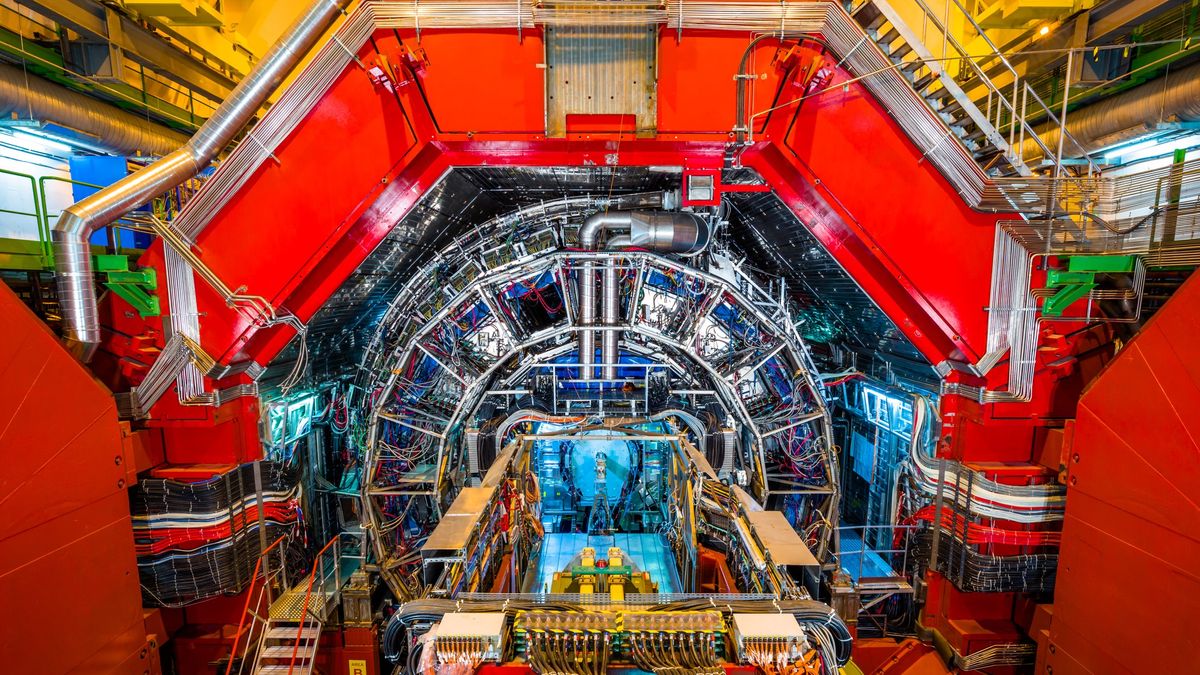
Ready, steady, go: the race to discover new physics returns today as the Large Hadron Collider (LHC) is re-ignited, firing heavy ion particles into one another at 99.99% the speed of light to recreate a state of primordial matter not seen since just after the Big Bang.
The Large Hadron Collider is the world's longest and most powerful particle accelerator, firing beams of subatomic particles around a 17-mile-long (27 kilometers) loop beneath the ground near Geneva, on the French-Swiss border. Since the LHC originally came online in 2010, its experiments have produced 3,000 scientific papers, with a range of findings including the most famous one of all: the discovery of the Higgs boson.
"It's really true to say that we have been making discoveries on a weekly basis," Chris Parkes, a spokesperson for the LHCb experiment, said at a press conference at the end of June.
New technology
The particle accelerator has spent the last three-and-a-half years receiving vital technological upgrades that will enable it to smash beams of particles with record energy of 6.8 trillion electronvolts (TeV) in collisions that will total an unprecedented 13.6 TeV. This is 4.6% higher than where it left off in October 2018.
An increased rate of particle collisions, improved ability to collect more data than ever before, and brand new experiments will pave the way for researchers to conduct science beyond the Higgs boson and, perhaps, even beyond the current Standard Model of particle physics.
In 2020 a new device, the Linear Accelerator (Linac) 4, was installed in the LHC. Rather than injecting protons into the system as before, Linac 4 will boost negatively charged hydrogen ions, which are protons accompanied by two electrons. As the ions move through Linac 4 the electrons are stripped away to leave just the protons, and interweaving these ions allows tighter bunches of protons to be formed. This results in narrower beams of protons being fired through the collider, increasing the rate of collisions.
Get the Space.com Newsletter
Breaking space news, the latest updates on rocket launches, skywatching events and more!
Perhaps the most important technological upgrade, however, is the system that triggers the experiments in the LHC to begin gathering data.
With scientific research now firmly in the era of big data, how to discern which data is worth recording and analyzing becomes an even bigger issue. "We have 14 million beam crossings per second," Parkes said. Each beam crossing sees bundles of particles crash into each other.
Previously, picking out the useful information from all those collisions was left to conventional hardware and the intuition of human researchers, resulting in only 10% of collisions inside the LHC being recorded. The new trigger system utilizes machine learning to more quickly analyze the situation and be more efficient at what data to collect for later analysis. This upgrade will, for example, see the LHCb triple its sampling rate, while the ALICE (A Large Ion Collider Experiment) instrument will increase its number of recorded events by a factor of 50.
"This is clearly a big deal," Luciano Musa, an ALICE spokesperson, said at the press conference.
New experiments
While there is still work to be done learning about the Higgs boson, the LHC is equipped to do much more besides that.
"We have this ambition of putting the Higgs boson into a broader context, and that simply can't be summarized in one or two questions," Gian Guidice, head of CERN's theoretical physics department, said during the press conference. "So we have a very wide program that addresses many questions in particle physics."
Two new detectors installed during the LHC's recent shutdown are FASER, the Forward Search Experiment, and SND, the Scattering and Neutrino Detector. FASER will search for light and weakly interacting particles, including neutrinos and possible dark matter, while SND will focus on neutrinos exclusively.
Neutrinos are elusive, ghost-like particles that barely interact with anything else around them — a bar of lead a light-year thick would only stop half the neutrinos passing through it — and trillions of them are passing harmlessly through your body each and every second. Given this, and despite scientists knowing that the collisions inside the LHC should regularly produce neutrinos, no neutrino created in a particle accelerator has ever been detected (the neutrinos observed by previous neutrino detectors mostly come from the sun). However, this is set to change, with FASER and SND expected to detect nearly 7,000 neutrino events between them over the next four years.
On the face of it, FASER and SND don't look like neutrino detectors. These tend to be enormous, such as the stainless steel tank of the Super Kamiokande detector in Japan that holds 50,000 metric tons of pure water, or the IceCube neutrino observatory at the South Pole, which has sensors placed in 0.6 cubic miles (one cubic kilometer) of ice beneath the surface. Instead, FASER is just 5 feet (1.5 meters) in length, and SND is only a little larger at 8 feet (2.4 meters). Rather than enormous volumes of fluid or ice, they feature simple tungsten detectors and films of emulsion, not dissimilar to old photographic film.
FASER and SND are able to get away with being so small because "the LHC produces a huge number of neutrinos, so you need less mass in the detector to get some of them to interact, and the neutrinos produced in the LHC's collisions are extremely high energy, and the probability of interaction goes up with energy," Jamie Boyd, a spokesperson for FASER, told Space.com.
FASER is located 1,500 feet (480 meters) downstream of the ATLAS experiment, in disused tunnels that were once part of the LHC's predecessor, the Large Electron-Positron Collider. The FASER and SND experiments are complementary — FASER is bang on the beamline, while SND is at an angle. This way, they are able to detect neutrinos of different energies coming from different particle collisions. Most neutrinos will pass unnoticed through the two experiments, but a small number will interact with the atoms in the dense tungsten layers, causing the neutrinos to decay and produce daughter particles that leave tracks in the emulsion called vertices that point back to the position of the interaction. Every three or four months the emulsion film is removed and sent to a laboratory in Japan for inspection. Already, a small prototype has detected neutrino candidates, but the prototype was too small to be able to confirm the measurements.
"The main result that we are looking for is what we call the cross-section," Boyd said. "This describes how, as a function of their energy, the three types of neutrino — electron, muon and tau neutrinos — interact."
These different types, or 'flavors', of neutrino, are able to oscillate into one another as they travel across large distances. For example, a neutrino might start out as a muon neutrino before oscillating into an electron neutrino. In the LHC, the distance between the neutrino detectors and the source of the collisions in the LHC is too small to expect any oscillations to take place unless there is a new particle involved.
"If we did see more electron neutrinos and less muon neutrinos than we expect, then that could indicate that there is an additional type of neutrino, called a sterile neutrino, that is causing these oscillations to take place," Boyd said. For now, sterile neutrinos remain hypothetical, and finding evidence for them would be a major discovery.
New theories
Speaking of discoveries, while the LHC was powered down for its most recent upgrades, data analysis from the old Tevatron particle accelerator at Fermilab in the U.S. that shut down in 2011 has turned up a tantalizing hint of physics operating beyond the Standard Model. Specifically, the Tevatron found evidence that the W boson particle, which is involved in mediating the weak force that governs radioactivity, could be more massive than the Standard Model predicts. Meanwhile, there have been curious readings from the LHC and the Tevatron of the behavior of electrons and muons that, if true, could defy predictions of the Standard Model. The onus is now on the LHC to investigate further.
However, scientists at the LHC are not willing to jump to conclusions about this or any other discrepancy from the Standard Model. Instead, they prefer to remain agnostic when it comes to various theories about what the LHC is observing, to avoid biasing the results.
"We are not running after theory," Fabiola Gianotti, the Director-General of CERN, said in the press conference. "I think our goal is to understand how nature works at the most fundamental level. Our goal is not to look for particular theories."
Chris Parkes is optimistic that the LHC can get to the bottom of these discrepancies, one way or the other. "We very much expect that from the new data that we collect, we can really probe these interesting hints that we have, and see whether they show any violations of the Standard Model," he said.
There's no rush. Following this new four-year observation run by the LHC, there will be another shutdown for further upgrades that will result in what's being referred to as the High Luminosity LHC. This will begin operating in about 2029, detecting more than 15 million Higgs bosons per year from collision energies of 14 TeV. Beyond the LHC, plans are afoot for a brand new accelerator at CERN called the Future Circular Collider (FCC), which will be powerful enough to reach energies of 100 TeV when it begins work around 2040. The FCC would be far larger than the LHC, with a tunnel 62 miles (100 km) long, although the concept has recently courted controversy with some physicists claiming that its possible $100 billion price tag would not be worth the benefits of building it and that the money could be more wisely spent on smaller, more focused projects.
That is all still in the future. In the here and now, the LHC still has Higgs bosons to create, neutrinos to detect, new particles to be found and theories to be put to the test. What new discoveries will we be talking about in four years' time?
Follow Keith Cooper on Twitter @21stCenturySETI. Follow us on Twitter @Spacedotcom and on Facebook.
Join our Space Forums to keep talking space on the latest missions, night sky and more! And if you have a news tip, correction or comment, let us know at: community@space.com.
Keith Cooper is a freelance science journalist and editor in the United Kingdom, and has a degree in physics and astrophysics from the University of Manchester. He's the author of "The Contact Paradox: Challenging Our Assumptions in the Search for Extraterrestrial Intelligence" (Bloomsbury Sigma, 2020) and has written articles on astronomy, space, physics and astrobiology for a multitude of magazines and websites.