The Large Hadron Collider reveals how far antimatter can travel through the Milky Way
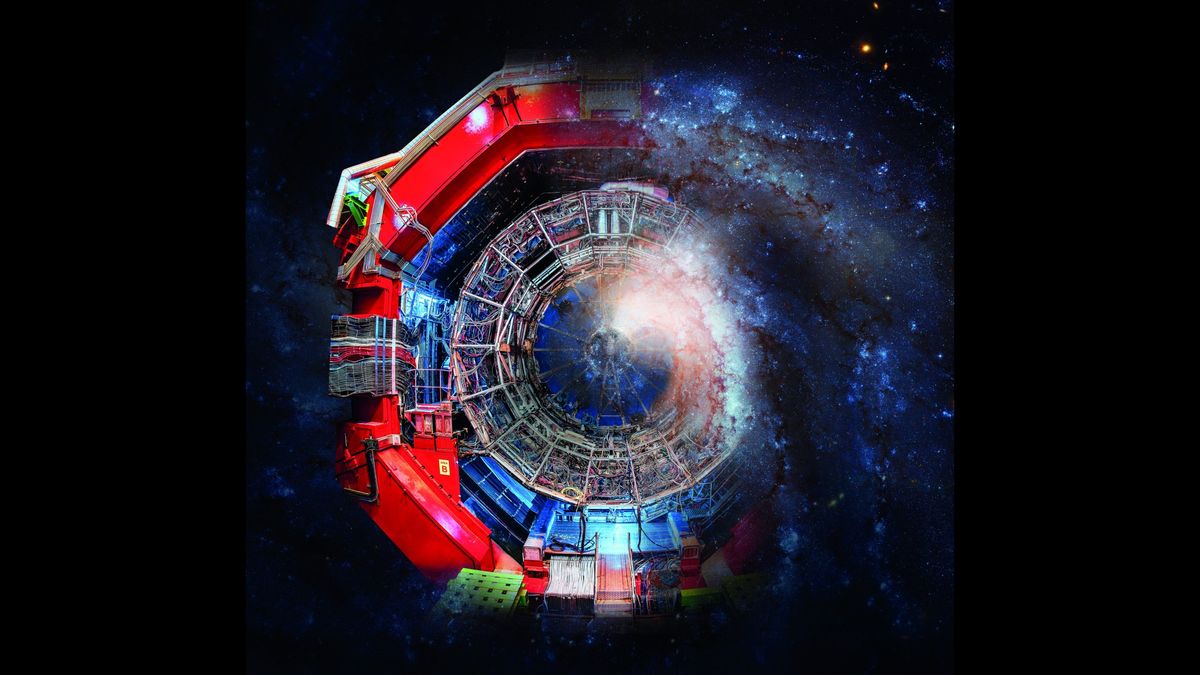
The antimatter counterparts of light atomic nuclei can travel vast distances through the Milky Way before being absorbed, new findings have revealed.
As these particles travel, they potentially act as "messengers" for dark matter, so the revelation could help astronomers in the hunt for dark matter, the mysterious substance that accounts for around 85% of the universe's total mass but remains invisible because it doesn't interact with light.
Scientists at the ALICE collaboration arrived at the finding using antihelium nuclei, the antimatter equivalent of helium nuclei, created by collisions of heavy atomic nuclei at the Large Hadron Collider (LHC).
"Our results show, for the first time on the basis of a direct absorption measurement, that antihelium-3 nuclei coming from as far as the center of our galaxy can reach near-Earth locations," ALICE physics coordinator Andrea Dainese, said in a statement.
Related: 10 cosmic mysteries the Large Hadron Collider could unravel
Although this form of antimatter can be created in particle accelerators like the LHC, there are no natural sources of antimatter nuclei or "antinuclei" on Earth. However, these anti-particles are produced naturally elsewhere in the Milky Way, with scientists favoring two possible origins.
The first suggested source for antinuclei is the interaction between high-energy cosmic radiation, which originates from outside the solar system, with atoms in the so-called interstellar medium that fills space between stars.
Get the Space.com Newsletter
Breaking space news, the latest updates on rocket launches, skywatching events and more!
The other suggested source of antinuclei is the annihilation of dark matter particles that are spread throughout the galaxy. While scientists know little about dark matter, they are certain that it is not comprised of particles like protons and neutrons that make up the everyday matter that forms stars, planets and us. Scientists believe dark matter, in contrast, is comprised of a wide range of particles with colorful names like WIMPs (weakly interacting massive particles) and MACHOs (massive compact halo objects). One scenario suggests that when dark matter particles collide, they annihilate into particles that then decay into light matter and antimatter particles, like electrons and their antimatter counterpart, positrons. If dark matter annihilation is indeed a source of antimatter in the universe, antimatter could point the way to dark matter, scientists hope.
Calculating the flux
The quest to learn more about dark matter has prompted the development of space-based missions such as the Alpha Magnetic Spectrometer (AMS) aboard the International Space Station (ISS). AMS was designed at CERN, the home of the LHC, to search the cosmos for light antimatter nuclei that could indicate the presence the mysterious dark matter.
But in order to determine whether dark matter is the source of antinucleons, scientists operating AMS and similar experiments first need to know how much light antimatter can pass through the Milky Way to reach their near-Earth locations, also known as the antiparticles' "flux."
This flux is dependent on several factors, including the antimatter source, the rate at which it produces antinuclei, and the rate at which the antinuclei disappear as they journey from the center of our galaxy to Earth. This disappearance occurs when antimatter particles meet particles of traditional matter; either both are annihilated or the antimatter is absorbed by the matter.
The ALICE Collaboration investigated the disappearance of antimatter by using the LHC to collide lead atoms that have been ionized, or stripped of electrons. The physicists then measured how antihelium-3 nuclei created by these collisions interact with normal matter in the form of the ALICE detector. The experiment revealed for the first time the rate at which antihelium-3 nuclei disappear as they encounter ordinary matter.
Using a computer program, the researchers then simulated the propagation of antiparticles through the galaxy and introduced to this model the disappearance rate measured at ALICE. This model allowed the researchers to extrapolate their results to the galaxy as a whole, and to look at the two suggested mechanisms of antinuclei production: One model assumed the antimatter came from cosmic-ray collisions with the interstellar medium, and the other model attributed antimatter to a hypothetical form of dark matter called weakly interacting massive particles (WIMPs).
For each of these mechanisms, the ALICE team estimated the transparency of the Milky Way to antihelium-3 nuclei — in other words, the distance antihelium-3 nuclei are free to travel before being destroyed or absorbed. The models revealed a transparency of around 50% in the dark matter model and a transparency ranging from 25% to 90% in the cosmic ray collision model, depending on the energy of the antinuclei created.
These values show that antihelium-3 nuclei originating from either process can travel long distances — up to several kiloparsecs, with each kiloparsec equivalent to around 3,300 light-years. (The Milky Way is about 30 kiloparsecs wide, according to NASA.)
The results could be important in future experiments that count how many antinuclei arrive around Earth and with what energies in hopes of determining whether the origin of these antiparticles is cosmic-ray collisions or dark matter annihilation.
"Our findings demonstrate that searches for light antimatter nuclei from outer space remain a powerful way to hunt for dark matter," ALICE spokesperson Luciano Musa said in the same statement.
The research is described in a paper published Monday (Dec. 12) in the journal Nature Physics.
Follow us on Twitter @Spacedotcom or on Facebook.
Join our Space Forums to keep talking space on the latest missions, night sky and more! And if you have a news tip, correction or comment, let us know at: community@space.com.
Robert Lea is a science journalist in the U.K. whose articles have been published in Physics World, New Scientist, Astronomy Magazine, All About Space, Newsweek and ZME Science. He also writes about science communication for Elsevier and the European Journal of Physics. Rob holds a bachelor of science degree in physics and astronomy from the U.K.’s Open University. Follow him on Twitter @sciencef1rst.
-
rod "The first suggested source for antinuclei is the interaction between high-energy cosmic radiation, which originates from outside the solar system, with atoms in the so-called interstellar medium that fills space between stars. The other suggested source of antinuclei is the annihilation of dark matter particles that are spread throughout the galaxy. While scientists know little about dark matter, they are certain that it is not comprised of particles like protons and neutrons that make up the everyday matter that forms stars, planets and us. Scientists believe dark matter, in contrast, is comprised of a wide range of particles with colorful names like WIMPs (weakly interacting massive particles) and MACHOs (massive compact halo objects). One scenario suggests that when dark matter particles collide, they annihilate into particles that then decay into light matter and antimatter particles, like electrons and their antimatter counterpart, positrons. If dark matter annihilation is indeed a source of antimatter in the universe, antimatter could point the way to dark matter, scientists hope."Reply
So, is DM confirmed in this report as to DM being a WIMP or MACHO? The paper, Measurement of anti-3He nuclei absorption in matter and impact on their propagation in the Galaxy | Nature Physics
States, "Propagation modellingThe possible sources of antinuclei in our Galaxy are either cosmic-ray interactions with nuclei in the interstellar gas or more exotic sources such as DM annihilations or decays. Cosmic rays mainly consist of protons and originate from supernovae remnants, whereas DM has so far escaped direct or indirect detection but its density profile can be modelled88."...Although coalescence-based models can successfully describe antinuclei production, the model uncertainties are still relatively large, which leads to substantial changes in the magnitude of antinuclei fluxes22,30,61."
Apparently, DM is not nailed down yet as to exactly what it is.