LIGO gravitational wave detector breaks 'quantum limit' to find deep universe black hole collisions
"We can now reach the deeper universe and are expecting to detect about 60 percent more mergers than before."
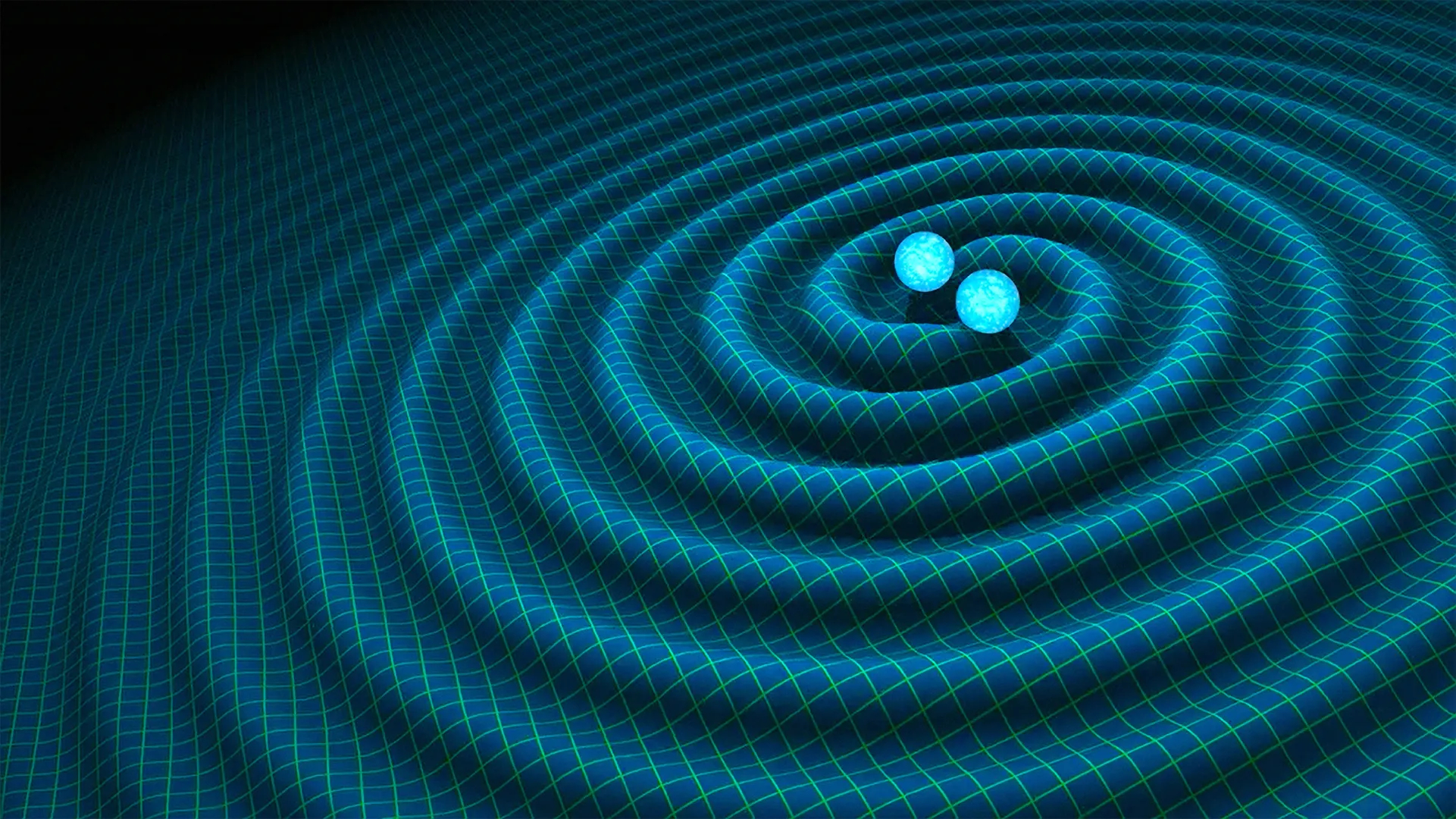
Earth’s premier gravitational wave detector just received a major upgrade that will significantly improve its ability to spot ripples in the fabric of space and time — undulations created by collisions between black holes or neutron stars, and sometimes, between both.
What this means is, during the next run of the Laser Interferometer Gravitational-Wave Observatory (LIGO), the instrument will be able to detect more merger events between these massive stellar remnants that form when enormous stars collapse at the end of their lives. LIGO will also be able to spot such impacts across greater distances thanks to the new upgrade, tracking gravitational waves that have rippled through spacetime for billions of years.
"We can now reach a deeper universe and are expected to detect about 60 percent more mergers than before," LIGO lab researcher, Wenxuan Jia, told Space.com. "LIGO will certainly detect farther-away binary coalescence events. With lower noise and higher signal-to-noise ratio, we can further constrain the parameters of the compact objects that merged together billions of years ago.
"The upgrade also increases our chances of detecting sub-stellar mass black holes in the universe. The latest experimental upgrade will benefit our detection of astrophysical signals in nearly every way."
Related: 2 merging supermassive black holes spotted at ‘cosmic noon’ in early universe
LIGO first became world-famous in Sept. 2015, when it detected gravitational waves from merging black holes for the first time. These ripples had traveled for around 1.4 billion years, squashing and squeezing spacetime as they made their way through the universe.
But since then, LIGO, and its partner gravitational wave detector Virgo, have detected signals from far more merging black hole pairs, colliding neutron stars, and mixed mergers between the two.
Get the Space.com Newsletter
Breaking space news, the latest updates on rocket launches, skywatching events and more!
LIGO researchers are particularly excited for the new upgrade, however, because this pushes the instrument beyond what is called the "quantum limit" — a first for a gravitational wave detector.
What is the 'quantum limit?'
LIGO is designed to measure an incredibly small change that can occur between lasers within two arms on the detector. The change occurs when gravitational waves ripple over those arms, Jia explained.
Basically, the instrument produces one laser and splits it into two beams that separately travel through the pair of 4-kilometer arms. The two beams then come into phase while traveling through the two arms, meaning they perfectly line up despite being in different places. Then, when the beams get reflected back via mirrors built into the device, they reunite and the peaks and troughs of the wavelengths meet. But here's where that length change comes in.
"The gravitational wave is a perturbation to spacetime," Jia explained. "When it propagates through the LIGO detector, it will change the length difference of the two 4-kilometer arms of LIGO as if it’s stretching one arm while shortening the other arm."
To be clear, the actual arms of LIGO aren't changing length. The lasers within them are — and this length change also forces the beams to increase in amplitude during the part when they reunite. So, in other words, the length difference resulting from the passage of gravitational waves leads to an amplitude change. And that causes the lasers' power to change as well.
However, because gravitational waves "squash and stretch" space, if they wash over these lasers, the length of the arms gets altered only by a very small amount so it's pretty hard to measure with standard mechanisms. But the effect is still observable thanks to the fact that light wavelength changes are reflected by the phase of the light. Remember the bit about both lasers lining up, being in the same phase? Well, a small change in arm length would mean that, when the light arrives back at the detector, it is no longer in phase — the peaks and troughs of the wavelength cancel each other out in a process called destructive interference.
There is another problem, however. The variations in length of these arms can sometimes get infinitesimally small — trillions of times smaller than a human hair, falling into sizes we see in the subatomic and quantum realms.
The weaker the gravitational wave, the less it changes the arm length and the smaller the effect on the laser. And when this effect gets really tiny, it bumps up against a principle in quantum physics called Heisenberg’s Uncertainty Principle, which says there is a limit to how precisely we can measure a correlated pair of physical quantities called "observables."
Practically, it means some gravitational waves have remained outside LIGO's abilities. But now, there seems to be a workaround.
"There exists a minimal uncertainty, or noise, when you want to measure the phase of a laser beam over time," Jia said. "A laser light has two observables, namely amplitude and frequency. We are doing a phase or frequency measurement with LIGO, and so we don’t care so much about the amplitude."
Jia added that Heisenberg’s Uncertainty Principle actually allows for a trade-off The team reduce the uncertainty of one observable they want — frequency — at the expense of increasing the uncertainty of the other one they do not — amplitude.
"We can reduce the frequency uncertainty by 'squeezing' the light. This clever idea allows us to surpass the minimal uncertainty, or the quantum limit, of the LIGO detector," Jia added.
"Since I was a young grad student, I’d heard about this kind of idea, but for 20 years, I didn’t think about it much. It seemed very ‘sci-fi' because, in school, you learn there’s a Heisenberg Uncertainty limit and you can only measure things so well before you reach that limit," Rana Adhikari, a professor of physics at the California Institute of Technology (Caltech) an told Space.com. "If you try to measure things more precisely, you disturb things. With this new upgrade, we’re able to basically measure as strongly as we want to. We can put full laser power into the system."
Adhikari added that his dream is to upgrade LIGO to the point at which it can detect gravitational waves at incredibly low sensitivities and, through this, access bigger black holes that may have existed in the very early universe. And the Caltech researcher also believes LIGO can be pushed even further in terms of sensitivity.
"Everything really depends on the quality of your materials. You can make really, really pure mirror materials, and then there’s almost no limit to how precisely you can measure things and push on the same technique, just doing it better and better over the years," Adhikari explained. "At that point, where the sensitivity has been upped by a factor of 10, the way that the LIGO works, you would be able to see black hole mergers from very, very early in the universe when the first galaxies were forming."
The achievement isn’t just a big deal for LIGO and the detection of gravitational waves; it also tells physicists that if you are willing to do a deal, it’s possible to surpass the quantum limit without violating the Uncertainty Principle.
"It's a very exciting event for me and the whole LIGO 'squeezing' team. A tremendous effort has been made to achieve this milestone over many years," Jia added. "It felt surreal to see the whole system working at first after we put together all subsystems carried out by many other teams. The success of frequency-dependent squeezing is not possible without such an awesome team and collaboration."
The team’s research was published in September in the journal Physical Review X.
Join our Space Forums to keep talking space on the latest missions, night sky and more! And if you have a news tip, correction or comment, let us know at: community@space.com.
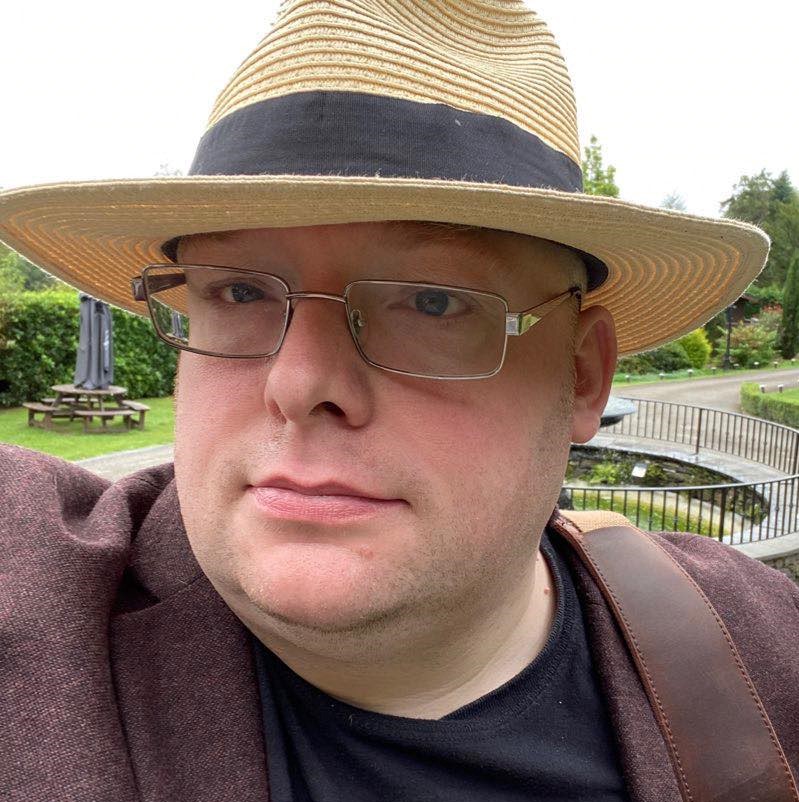
Robert Lea is a science journalist in the U.K. whose articles have been published in Physics World, New Scientist, Astronomy Magazine, All About Space, Newsweek and ZME Science. He also writes about science communication for Elsevier and the European Journal of Physics. Rob holds a bachelor of science degree in physics and astronomy from the U.K.’s Open University. Follow him on Twitter @sciencef1rst.
-
Classical Motion "The gravitational wave is a perturbation to spacetime," Jia explained. "When it propagates through the LIGO detector, it will change the length difference of the two 4-kilometer arms of LIGO as if it’s stretching one arm while shortening the other arm."Reply
"To be clear, the actual arms of LIGO aren't changing length. The lasers within them are — and this length change also forces the beams to increase in amplitude during the part when they reunite. So, in other words, the length difference resulting from the passage of gravitational waves leads to an amplitude change. And that causes the lasers' power to change as well."
Which is it?
What is the principle of detection? -
Torbjorn Larsson
Elegant! I knew they were working on improvements but I had no idea that they were going to take the squeezing further.Admin said:The sensitivity of LIGO has squeezed the quantum limit, meaning it can now detect merging black holes and neutron stars on smaller scales and at greater distances than ever before.
LIGO gravitational wave detector breaks 'quantum limit' to find deep universe black hole collisions : Read more -
Torbjorn Larsson
It seems poorly written. I think the article wants to point out that the arm lengths are not always changed in totality as the gravitational wave pass (depending on the sizes) and definitely not after wave passed. But in all cases that the photon travel distances will change.Classical Motion said:"The gravitational wave is a perturbation to spacetime," Jia explained. "When it propagates through the LIGO detector, it will change the length difference of the two 4-kilometer arms of LIGO as if it’s stretching one arm while shortening the other arm."
"To be clear, the actual arms of LIGO aren't changing length. The lasers within them are — and this length change also forces the beams to increase in amplitude during the part when they reunite. So, in other words, the length difference resulting from the passage of gravitational waves leads to an amplitude change. And that causes the lasers' power to change as well."
Which is it?
What is the principle of detection?
The amplitude description may refer to the interference detection.
Here is LIGO's own description:
Gravitational waves cause space itself to stretch in one direction and simultaneously compress in a perpendicular direction. In LIGO, this causes one arm of the interferometer to get longer while the other gets shorter, then vice versa, back and forth as long as the wave is passing. The technical term for this motion is "Differential Arm" motion, or differential displacement, since the arms are simultaneously changing lengths in opposing ways.
https://www.ligo.caltech.edu/page/what-is-interferometer
As described above, as the lengths of the arms change, so too does the distance traveled by each laser beam. A beam in a shorter arm will return to the beam splitter before a beam in a longer arm--as the wave passes, each arm oscillates between being the shorter arm and the longer arm. When they arrive back at the beamsplitter (where they re-merge), the light waves no longer meet up nicely; they are out of phase. Instead, they shift in and out of alignment for as long as the wave is passing. In simple terms, this shifting alignment results in a flicker of light emerging from the interferometer. -
Classical Motion Thanks, that's what I had read.Reply
How's come the LIGO light is not effected by the compression and expansion of space.........but the redshift of stars is? With current theory, can we trust this dynamic?
I believe the whole setup is poorly designed, for they are using a flux, full of phase and noise. Why not use radio....and use sequentially spaced photons? Remove that flux. These longer photons are much quieter and the duration is long enough to measure much more exact phase differences.
What are the frequencies of the g waves that have been detected? They are low frequency if I recall.
And I wonder if there is any temperature flutter from it. Does it need to be constantly corrected and tuned?