
Nuclear rockets could travel to Mars in half the time − but designing the reactors that would power them isn't easy
Fission technology is well established in power generation and nuclear-powered submarines, and its application to drive or power a rocket could one day give NASA a faster, more powerful alternative to chemically driven rockets.
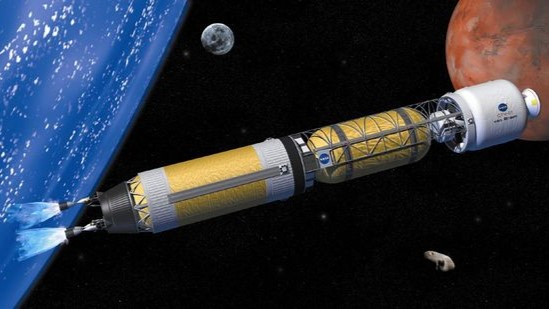
This article was originally published at The Conversation. The publication contributed the article to Space.com's Expert Voices: Op-Ed & Insights.
Dan Kotlyar is an Associate Professor of Nuclear and Radiological Engineering at the Georgia Institute of Technology.
NASA plans to send crewed missions to Mars over the next decade – but the 140 million-mile (225 million-kilometer) journey to the red planet could take several months to years round trip.
This relatively long transit time is a result of the use of traditional chemical rocket fuel. An alternative technology to the chemically propelled rockets the agency develops now is called nuclear thermal propulsion, which uses nuclear fission and could one day power a rocket that makes the trip in just half the time.
Nuclear fission involves harvesting the incredible amount of energy released when an atom is split by a neutron. This reaction is known as a fission reaction. Fission technology is well established in power generation and nuclear-powered submarines, and its application to drive or power a rocket could one day give NASA a faster, more powerful alternative to chemically driven rockets.
NASA and the Defense Advanced Research Projects Agency are jointly developing NTP technology. They plan to deploy and demonstrate the capabilities of a prototype system in space in 2027 – potentially making it one of the first of its kind to be built and operated by the U.S.
Related: NASA, DARPA to launch nuclear rocket to orbit by early 2026
Breaking space news, the latest updates on rocket launches, skywatching events and more!
Nuclear thermal propulsion could also one day power maneuverable space platforms that would protect American satellites in and beyond Earth’s orbit. But the technology is still in development.
I am an associate professor of nuclear engineering at the Georgia Institute of Technology whose research group builds models and simulations to improve and optimize designs for nuclear thermal propulsion systems. My hope and passion is to assist in designing the nuclear thermal propulsion engine that will take a crewed mission to Mars.
Nuclear versus chemical propulsion
Conventional chemical propulsion systems use a chemical reaction involving a light propellant, such as hydrogen, and an oxidizer. When mixed together, these two ignite, which results in propellant exiting the nozzle very quickly to propel the rocket.
These systems do not require any sort of ignition system, so they’re reliable. But these rockets must carry oxygen with them into space, which can weigh them down. Unlike chemical propulsion systems, nuclear thermal propulsion systems rely on nuclear fission reactions to heat the propellant that is then expelled from the nozzle to create the driving force or thrust.
In many fission reactions, researchers send a neutron toward a lighter isotope of uranium, uranium-235. The uranium absorbs the neutron, creating uranium-236. The uranium-236 then splits into two fragments – the fission products – and the reaction emits some assorted particles.
More than 400 nuclear power reactors in operation around the world currently use nuclear fission technology. The majority of these nuclear power reactors in operation are light water reactors. These fission reactors use water to slow down the neutrons and to absorb and transfer heat. The water can create steam directly in the core or in a steam generator, which drives a turbine to produce electricity.
Nuclear thermal propulsion systems operate in a similar way, but they use a different nuclear fuel that has more uranium-235. They also operate at a much higher temperature, which makes them extremely powerful and compact. Nuclear thermal propulsion systems have about 10 times more power density than a traditional light water reactor.
Nuclear propulsion could have a leg up on chemical propulsion for a few reasons.
Nuclear propulsion would expel propellant from the engine’s nozzle very quickly, generating high thrust. This high thrust allows the rocket to accelerate faster.
These systems also have a high specific impulse. Specific impulse measures how efficiently the propellant is used to generate thrust. Nuclear thermal propulsion systems have roughly twice the specific impulse of chemical rockets, which means they could cut the travel time by a factor of 2.
Nuclear thermal propulsion history
For decades, the U.S. government has funded the development of nuclear thermal propulsion technology. Between 1955 and 1973, programs at NASA, General Electric and Argonne National Laboratories produced and ground-tested 20 nuclear thermal propulsion engines.
But these pre-1973 designs relied on highly enriched uranium fuel. This fuel is no longer used because of its proliferation dangers, or dangers that have to do with the spread of nuclear material and technology.
The Global Threat Reduction Initiative, launched by the Department of Energy and National Nuclear Security Administration, aims to convert many of the research reactors employing highly enriched uranium fuel to high-assay, low-enriched uranium, or HALEU, fuel.
High-assay, low- enriched uranium fuel has less material capable of undergoing a fission reaction, compared with highly enriched uranium fuel. So, the rockets needs to have more HALEU fuel loaded on, which makes the engine heavier. To solve this issue, researchers are looking into special materials that would use fuel more efficiently in these reactors.
NASA and the DARPA’s Demonstration Rocket for Agile Cislunar Operations, or DRACO, program intends to use this high-assay, low-enriched uranium fuel in its nuclear thermal propulsion engine. The program plans to launch its rocket in 2027.
As part of the DRACO program, the aerospace company Lockheed Martin has partnered with BWX Technologies to develop the reactor and fuel designs.
The nuclear thermal propulsion engines in development by these groups will need to comply with specific performance and safety standards. They’ll need to have a core that can operate for the duration of the mission and perform the necessary maneuvers for a fast trip to Mars.
Ideally, the engine should be able to produce high specific impulse, while also satisfying the high thrust and low engine mass requirements.
Ongoing research
Before engineers can design an engine that satisfies all these standards, they need to start with models and simulations. These models help researchers, such as those in my group, understand how the engine would handle starting up and shutting down. These are operations that require quick, massive temperature and pressure changes.
The nuclear thermal propulsion engine will differ from all existing fission power systems, so engineers will need to build software tools that work with this new engine.
My group designs and analyzes nuclear thermal propulsion reactors using models. We model these complex reactor systems to see how things such as temperature changes may affect the reactor and the rocket’s safety. But simulating these effects can take a lot of expensive computing power.
We’ve been working to develop new computational tools that model how these reactors act while they’re starting up and operated without using as much computing power.
My colleagues and I hope this research can one day help develop models that could autonomously control the rocket.
Join our Space Forums to keep talking space on the latest missions, night sky and more! And if you have a news tip, correction or comment, let us know at: community@space.com.
Dr. Dan Kotlyar is an Assistant Professor in the Nuclear and Radiological Engineering, G.W.W. School of Mechanical Engineering. He received his B.Sc. in Engineering in 2008, MSc in Nuclear Engineering in 2010, and PhD in Nuclear Engineering in 2013 from Ben-Gurion University, Israel. In 2014, he joined the University of Cambridge as a Research Associate in the Engineering Design Center. In 2014, he was elected as a Research Fellow at Jesus College. He is the recipient of the NRC Faculty Development Fellowship. Dr. Kotlyar’s research interests include development of numerical methods and algorithms for coupled Monte Carlo, fuel depletion and thermal hydraulic codes. In particular, he specializes in applying these methods to the analysis of advanced reactor systems. Dr. Kotlyar’s research also focuses on optimizing the performance of various fuel cycles in terms of fuel utilization, proliferation, and cost. Dr. Kotlyar profoundly believes in education through research and thus integrates practical reactor system design into his lectures.
-
Unclear Engineer It would be a lot easier if they just went ahead and used highly enriched uranium for the reactor. These reactors are not going to be used where they could be stolen by non-nuclear states so that they could lead to "proliferation" of atomic weapons in currently non-nuclear countries.Reply
And, anyway, the countries like North Korea and Iran are developing nuclear weapons anyway, as did India and Pakistan.
So, I do not see any realistic reason to not use the best possible materials for space exploration vehicles. We aren't going to return these thing to Earth's surface once they have gone critical and produced power and radioactive byproducts. -
Passacaglia Most likely reaction mass will be hydrogen (as shown in the diagram) and not oxygen (as stated in the text).Reply -
newtons_laws
Your argument has a lot of merit. I believe the HALEU fuel being considered has about 20% U235 enrichment.Unclear Engineer said:It would be a lot easier if they just went ahead and used highly enriched uranium for the reactor. These reactors are not going to be used where they could be stolen by non-nuclear states so that they could lead to "proliferation" of atomic weapons in currently non-nuclear countries.
And, anyway, the countries like North Korea and Iran are developing nuclear weapons anyway, as did India and Pakistan.
So, I do not see any realistic reason to not use the best possible materials for space exploration vehicles. We aren't going to return these thing to Earth's surface once they have gone critical and produced power and radioactive byproducts. -
fj.torres The time for nuclear thermal was last century.Reply
By the time the politics align to put fission reactors into space (2040?) newer tech will be viable, ranging from MHD-enhanced exhaust to plasma engines. Like a VASIMR derivative driven by a reactor instead of solar or fuel cell. And hydrogen is finicky and low mass. Better exhaust materials can be found, especially if lunar sourced. In fact, if He3 is actually mine-able on the moon, a fusion drive might be economically viable.
Where fission will be most useful is in providing electrical power for the truly advanced drives. Say something like the Westinghouse 5MW eVinci. And that is still a thermal-based tech. If any of the small fusion power concepts do pan out, even more exotic options would be doable.
It may be best to stop trying to undo the mistakes and bad choices of the past and look instead to the technologies that are emerging for both power and propulsion...
...while remembering there will still be a need for cheap chemical vehicles for landers and such. It is not too fanciful to conceive of a large advanced transfer vehicle with a dozen Starship-class ships attached to hold cargo and passengers.
Fast propulsion systems are necessary but you have to get off the mudball first and most advanced concepts are unlikely to provide the thrust needed for that. Until the ivory tower designs can get off planet on their own there will still be a need for chemical. -
Meteoric Marmot I believe the issue with enhanced uranium is that it violates weapons treaties and can't be produced. I'm going from memory here, so I could be wrong.Reply -
billslugg The permanent members of the Security Council can enhance uranium, no one else who signed NNPT can. The five are obligated to provide the signatories with reactor fuel. Those countries did not sign NNPT, which is Israel, Pakistan, India and South Sudan can do whatever they want but they can also be "first targeted" legally.Reply -
bolide
The 'proliferation' problem is not by any means limited to non-nuclear states trying to produce nuclear bombs. The stuff still has to be produced, handled and transported on the ground. Any sort of bad actor gaining any sort of control over any amount of HEU could create catastrophic risk.Unclear Engineer said:It would be a lot easier if they just went ahead and used highly enriched uranium for the reactor. These reactors are not going to be used where they could be stolen by non-nuclear states so that they could lead to "proliferation" of atomic weapons in currently non-nuclear countries.
And, anyway, the countries like North Korea and Iran are developing nuclear weapons anyway, as did India and Pakistan.
So, I do not see any realistic reason to not use the best possible materials for space exploration vehicles. We aren't going to return these thing to Earth's surface once they have gone critical and produced power and radioactive byproducts. -
bolide I wonder why they choose the lightest possible substance for the propellant. Something heavier should provide more thrust for a given exhaust velocity, or require a lesser velocity for the same thrust. It would also take up less space.Reply -
Classical Motion I have read some unbelievable things about metal foams. Like that within the same volume, a foam can hold more molecules than a vacant volume under our highest tank pressures.Reply
And the molecules are under very low pressure. And the foam under very low tenser stress.
That’s pretty amazing.
The big breakthroughs in the future will be with structure, not mass and energy.
The conversion and ratio of mass and energy is set and varied with a physical structure. -
Unclear Engineer A coupe of comments:Reply
1. The countries with nuclear weapons have the capabilities and rights under the treaty to produce weapons-grade enriched uranium. And they all need to continue to do so to maintain their nuclear warheads, which have continuous natural nuclear decay going on that can degrade them. So, the issue is commercial use of weapons-grade material, not having it, and treaties against "weapons in space", which a rocket motor really is not.
2. Regarding using light weight atoms (or molecules) for the highest efficiency in producing rocket thrust, the reason is that rocket thrust is based on the momentum of the exhaust gas, while the speed of the exhaust gas depends on the amount of energy that can be put into it.
With a nuclear rocket, the energy is not dependent on the chemistry of the propellants, so the choice of exhaust gases is free of the need for it to be heated by burning it.
So, for example, if you apply enough energy to push an atom with atomic mass 1 to a velocity of 1, that amount of energy is 1/2 x mass x velocity squared which is 0.5 for those values.
Now consider a single exhaust gas molecule with a mass of 2. Assuming the same amount of energy in that exhaust particle and solving for its velocity, that particle will have a velocity equal to the square root of (0.5 divided by1/2 and divide by 2) = square root of 1/2 = 0.71
Now, look at the momentum for the same amount of energy in the two particles of different masses. Momentum is proportional to the velocity, not the square of the velocity.
So, the particle with mass 1 and velocity 1 has momentum of 1, while the particle with mass 2 has momentum equal to 2 times 0.71 = 1.42. So, it seems like the heavier particle provides more thrust for a given amount of energy per particle. But, not for a given mass of propellant, which is the concern for rocket vehicle performance.
The efficiency ("specific impulse" abbreviated Isp) of a rocket motor is the amount of thrust per unit mass of the propellant used per unit time. So for the particle with mass 1 being used one per second, the Isp is 1.0 divided by one per second, which equals 1 second. The particle with mass 2 being used 1 per second has an Isp of 1.42 divided by 2 equals 0.71. so, the efficiency of the heavier particle is lower by 29%.
To really appreciate the significance of the Isp efficiency, think of it as the total amount of "impulse" that it can produce, which is the amount of force multiplied by the length of time it can be produced. So, for the 2 propellant particles of different masses, the lighter particle with an Isp of 1 second would produce a total "impulse" of 1 times 10 =10 for 10 seconds of propellant flow, while the heavier particle with the same mass flow rate would only produce 0.71 times 10 = 7.1 , because the same mass flow rate of the heavier propellant is 1/2 the particle flow rate of the lighter propellant.
So, for a given total mass of propellant, the lighter exhaust particles provide more total thrust over the same period of time that the rocket engine runs.
That is the basic physics, but the engineering is not so simple. For one thing, the weight of the equipment needed to create the propellant exhaust velocity is much different between a chemical rocket and a nuclear thermal rocket, which needs a whole, heavy nuclear reactor and probably some shielding that a chemical rocket does not have. And, there are also some other issues about where the energy from the reactor can go besides the velocity of the propellant particles. For instance, if the propellant particle is a molecule instead of a single atom, when it is heated, some of the energy goes into either vibrational and rotational states of the atoms within the molecule, or into separation of those atoms by breaking the chemical bond between them.
So, in reality, the benefits of a nuclear rocket vs a chemical rocket need to be calculated between 2 actual designs, one for each, to determine the real difference for specific mission requirements.