Protons: The essential building blocks of atoms
Protons are tiny particles just a femtometer across, but without them, atoms wouldn't exist.
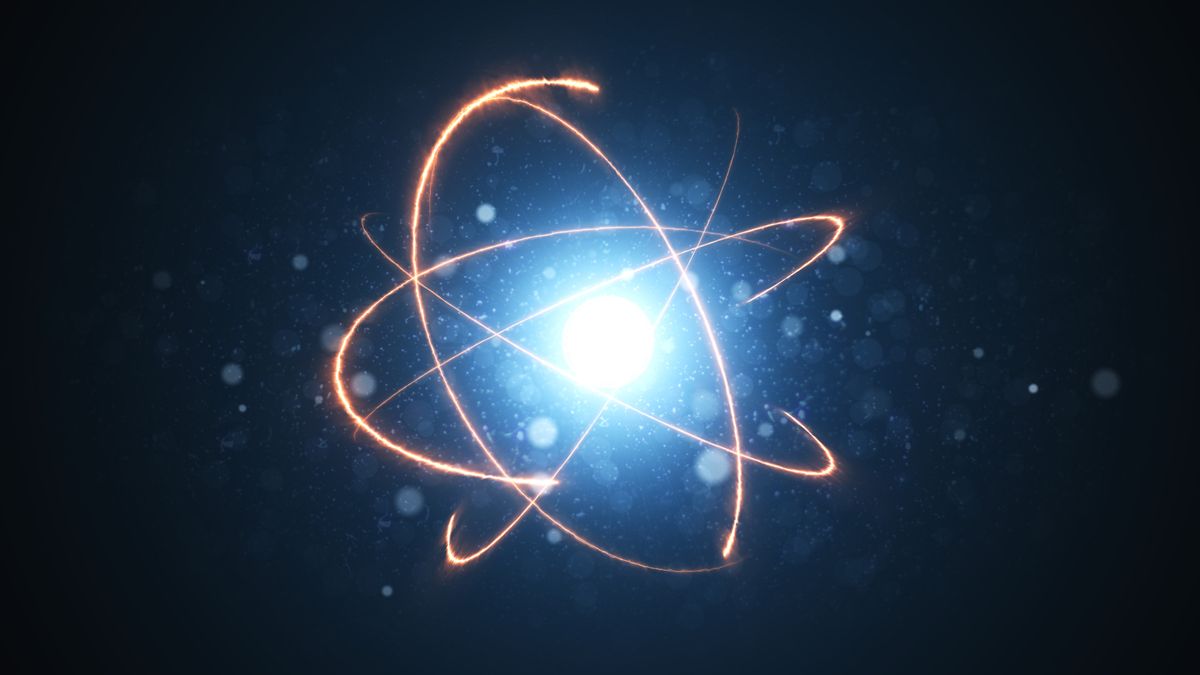
Protons are tiny subatomic particles that, along with neutrons, form the nucleus of an atom.
The heavier the atom, the more protons (and neutrons) it contains. Hydrogen, which is the lightest element, has a nucleus made from a single proton. The heaviest element in the Periodic Table, which is Oganesson, has 118 protons.
Protons are not elementary particles; they're actually made up of even smaller particles called quarks. Like neutrons, protons contain three quarks (two "up" quarks and one "down" quark) that are held together inside a proton by the Strong Force. Particles made of three quarks are referred to as "baryons", hence when physicists refer to "baryonic matter" they are specifically referring to matter made from protons and neutrons that make up atoms that then build all the people, planets, stars, galaxies and everything else that we can visibly see in the universe around us.
Discovery of protons
Throughout most of the 19th century, it was thought that atoms were the smallest and most basic building block of all matter, but as that century neared its end, the evidence that atoms are actually made of smaller particles began to grow. Scientists began to experiment with anode and cathode rays — these are positively and negatively charged beams produced by gas discharge tubes.
In 1897 J. J. Thomson discovered that cathode rays are streams of electrically-negative subatomic particles called electrons, which were being liberated from the atoms in the discharge tube. Correspondingly, anode rays must be streams of ions, which are positively charged atoms. In particular, hydrogen ions were recognized in anode rays in 1898 by the German physicist Wilhelm Wien.
The first hypothesis of the structure of atoms, therefore, had negatively charged electrons spread through an amorphously distributed mass of positive charge. It was called the plum pudding model, with the electrons being made analogous to plums embedded in dough.
The British physicist Ernest Rutherford was dubious of this model. Between 1909 and 1911 Hans Geiger and Ernest Marsden, under the tutelage of Rutherford at the University of Manchester, fired what were called alpha particles — what we know today as helium nuclei — at a leaf of gold foil. In the plum pudding model, the alpha particles should have just passed straight through the gold atoms, or been deflected a little bit.
Instead, Geiger and Marsden found in their experiment that sometimes the alpha particles were deflected at large angles, or even bounced straight back. That could only happen if there were a knot of electric charge at the center of an atom, rather than being spread out as in the plum pudding model. This convinced Rutherford that atoms actually consisted of a tiny, tight nucleus surrounded by empty space with electrons orbiting around the nucleus at a distance.
This model, albeit simplified because it doesn't incorporate the quantum mechanical behavior of the electrons, is referred to as the Bohr model after Niels Bohr, who along with Rutherford put all the pieces together.
In the gold leaf experiment, the deflected alpha particles were encountering this nucleus. But what was the nucleus made of?
Various experiments, including some performed by Rutherford, showed that hydrogen nuclei could come out of other elements, and by 1920 Rutherford had figured that hydrogen nuclei must be the basic building block of all atomic nuclei since hydrogen is the lightest element. He called the hydrogen nucleus a proton, meaning "first" in Greek because Rutherford saw it as the first building block for all atoms. Today we know that protons (and neutrons) are formed from even smaller particles, quarks, and that the nucleus of an atom is made from protons and neutrons (with the exception of the basic form of hydrogen, which has no neutrons).
What is the charge of a proton?
A proton has what is called an "elementary charge", or "e" in shorthand. It is the basic unit of charge against which all other charges are measured. Only quarks have a smaller charge, being a third or two-thirds of the elementary charge.
The elementary charge of the proton is 1.602192 x 10^–19 coulombs (C). This is the exact equal and opposite of the charge of an electron, which is — 1.602192 x 10^-19 coulombs. Because their charges are equal, and because the other co-inhabitant of the atomic nucleus, the neutron, is neutral, then so long as the number of protons and electrons are equal then their charges cancel out and atoms are electrically neutral. Remove an electron from around an atom, however, and this upsets the balance between the cumulative charges of the electrons and the protons, and the atom becomes positively charged — an ion.
What is the size and mass of a proton?
Given that protons are subatomic particles at the very heart of an atom, they are therefore extremely small, measuring just hundreds of trillionths of a meter (10^–15 meters). Robert Hofstadter, who was an American physicist who won the Nobel Prize for his work characterizing protons and neutrons, described this scale of 10^–15 meters as a 'femtometer', named after the famous physicist Enrico Fermi.
Particle | Mass (kg) | Radius (m) | Charge (C) |
---|---|---|---|
Proton | 1.673 x 10^–27 | 0.83 x 10^–15 | 1.6021 x 10^–19 |
Neutron | 1.674 x 10^–27 | 0.84 x 10^–15 | No charge |
Electron | 9.109 x 10^–31 | 10^–18-10^–22 | –1.6021 x 10^–19 |
We can compare the scale of a femtometer to the width of a human hair, which is in the region of a hundred millionths, or 10^–8, of a meter, or the radius of an entire atom about ten billionths, or 10^–10, of a meter.
Given their tiny size, they also have a tiny mass, just 1.673 x 10^–27 kilograms. That's a thousand trillion trillionths of a kilogram. For comparisons, it's 1,836 times more massive than an electron (9.1 x 10^–31 kilograms). It's also just slightly less massive than a neutron (1.674 x 10^–27 kilograms, or 1.008 times more massive than a proton).
Protons in space
Given that hydrogen is by far the most common element (or molecule) in the universe, and since hydrogen nuclei are just single protons, then suffice it to say the science of protons can teach us much about the distribution of matter and the violent mechanisms that drive some of the most energetic phenomena in the cosmos.
Star-forming nebulae filled with hydrogen gas in deep space are often referred to as H-II regions. This notation means that the hydrogen has been ionized by the ultraviolet light from young stars around it (H-I is neutral atomic hydrogen; H-II is ionized); the energy of the ultraviolet photon that the hydrogen absorbs is enough to kick out the electron. Since a hydrogen atom consists of just a single proton and a single electron, losing the electron leaves just the proton. When a proton in the nebula recaptures an electron, it emits a photon of light at a characteristic wavelength of 656.3 nanometers, known as H-II emission.
Protons are also vital in the core of the sun, where the energy that manifests as the light and heat of the sun is generated via a mechanism known as the proton-proton chain. In the core of the sun, the temperature reaches 27 million degrees Fahrenheit (15 million degrees Celsius) — sufficient for nuclear fusion. In these high temperatures, all atoms are ionized, and since the sun is mainly hydrogen, then this means that the core of the sun is filled with protons.
Related: Proton fusion, the sun's power source, explained (infographic)
In the proton-proton chain, two protons coming together in these conditions at the center of the sun can fuse, in the process giving off a neutrino and a positively charged positron (which is the antimatter equivalent of an electron).
Losing the positive charge turns one of the protons into a neutral neutron, and together the proton and neutron form deuterium (an isotope of hydrogen). This deuterium nucleus can then fuse with another proton, forming helium-3 (made of two protons and a neutron) and emitting energy in the process that eventually winds its way to the surface of the sun as radiation, which we see as light and feel as heat.
Meanwhile, the helium-3 nucleus can then fuse with another helium-3 nucleus formed through the same process, creating helium-4 (2 protons, 2 neutrons) and emitting two other protons. These other protons can then go on to form more helium-3 and so on in a chain reaction, releasing more energy in the process. The sun contains enough hydrogen nuclei to continue doing this for another 5 billion years.
The solar wind, which is a flow of charged particles streaming away from the sun's atmosphere, includes copious protons besides electrons and various atomic nuclei. When the solar wind collides with a planetary atmosphere such as that of Earth, the protons and electrons ride magnetic-field lines down towards the poles of the planet, interacting and ionizing atoms and molecules in the atmosphere. These atoms and molecules then glow, producing the auroral displays of the Northern and Southern Lights.
Sometimes, the sun will erupt in a solar flare, often resulting in the release of a coronal mass ejection. These violent solar eruptions can accelerate protons to high energies. Such "solar energetic particles" are pushed up to almost the speed of light as they race away from the sun, and are a radiation hazard to astronauts and passengers in high-altitude airliners.
There are also high-energy protons (and alpha particles) coming from beyond our solar system. These "cosmic rays" pack a punch, traveling a smidgen below the speed of light, but their origin remains a perplexing mystery. Evidently, they are accelerated by powerful magnetic fields, and prime suspects include active galactic nuclei and the black hole environments they contain. Alternatively, supernova remnants and dense star-forming regions have also been proposed as origin points for these particle bullets sent our way.
Follow Keith Cooper on Twitter @21stCenturySETI. Follow us on Twitter @Spacedotcom and on Facebook.
Additional resources
Learn about how complicated the proton is with this article from Quanta Magazine. Explore how recent measurements have narrowed down the size of the proton with this Nature article. Read more about protons and learn about nuclear fusion with the help of some handy diagrams in this article from The University of Warwick.
Bibliography
Particle Physics, by Brian R. Martin (2011, One-World Publications)
Collins Internet-Linked Dictionary of Physics (2007, Collins)
Join our Space Forums to keep talking space on the latest missions, night sky and more! And if you have a news tip, correction or comment, let us know at: community@space.com.
Get the Space.com Newsletter
Breaking space news, the latest updates on rocket launches, skywatching events and more!
Keith Cooper is a freelance science journalist and editor in the United Kingdom, and has a degree in physics and astrophysics from the University of Manchester. He's the author of "The Contact Paradox: Challenging Our Assumptions in the Search for Extraterrestrial Intelligence" (Bloomsbury Sigma, 2020) and has written articles on astronomy, space, physics and astrobiology for a multitude of magazines and websites.
-
rod "Protons, Neutrons and Electrons"Reply
My observation, a useful table here showing the particle, mass in kg, radius in meters, and charge in coulombs. The radius of the proton is 0.83 x 10^–15 m, the radius of the electron is 10^–18-10^–22 m. An interesting report but how does the BB cosmology explain the origin of the proton, neutron, and electron in the table, consider dark matter, dark energy, and the quark-gluon plasma phase. The exotic physics of inflation and BB cosmology is now working at or near the Planck time and Planck length, 10^20 magnitude smaller than the size of a proton. Consider the period in the BB model from Planck time and Planck length of universe size at the beginning and when the CMBR appears as light, the universe radius is perhaps 40-41 million light years.
Who, what, when, where, how, and why investigative reporting is needed in the BB model.
Here are some recent reports asking these same questions about the BB cosmology.
https://forums.space.com/threads/quarks-what-are-they.58460/
https://forums.space.com/threads/atoms-what-are-they-and-how-do-they-build-the-elements.58433/
https://forums.space.com/threads/what-makes-newtons-laws-work-heres-the-simple-trick.57302/ -
Unclear Engineer The thing that seems to be missing in all of these (highly repetitive) simplified descriptions of "fundamental particles" is that the protons and neutrons are supposed to be made of "fundamental" particles called quarks, but, somehow, a neutron can convert to a proton plus a lepton (electron), and leptons are not made of quarks. See https://en.wikipedia.org/wiki/Free_neutron_decay .Reply
So, if an "up quark" can become a "down quark" by emitting an electron and an antineutrino, thereby changing its charge and mass, it sure looks like there is something more "fundamental" than quarks, which would also includes the makings of the leptons. See https://en.wikipedia.org/wiki/Preon . Preons, also called "partons" do not seem to currently be in-favor with theorists. The Higgs boson seems to be their answer to why partons are unnecessary. But, the models are still "incomplete" (as in don't explain everything).
Whatever, these "explanations" about "quarks" seem to leave a gaping hole in the understanding they seem to want to convey.