Our universe's smallest galaxies hold the largest star factories. Here's why
It's possible not enough of their stars explode in supernovas.
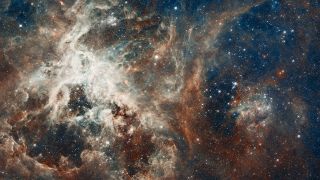
Some of the biggest, most intense regions of star formation are found in the smallest of galaxies, and scientists believe this is because stars reaching the ends of their lives in the so-called dwarf galaxies are more likely to turn into black holes than explode in supernovas. The contrast is large enough, the team says, that dwarf galaxies experience a 10-million-year delay in blowing all their star-forming material away, a process usually dependent on the forces of supernovas.
In other words, dwarf galaxies are able to hang onto their precious trove of star-forming molecular gas for longer, allowing star-forming regions to grow in size and intensity, and produce more stars.
Examples of such huge star-forming regions in local dwarf galaxies include 30 Doradus (the Tarantula Nebula) in the Large Magellanic Cloud, located just about 160,000 light-years away, and Markarian 71 in the galaxy NGC 2366, located about 10 million light years away.
Star-forming regions can produce stars of all masses; they mostly yield smaller stars, but create a handful of massive stars, too. When these massive stars reach the end of their life after a few million years, their cores collapse to either form a neutron star or a stellar-mass black hole. In the former scenario, a star's outer layers rebound off the neutron star and explode as a supernova. In the latter case, however, almost an entire star falls into the resultant black hole with nary a whimper.
"As stars go supernova, they pollute their environment by producing and releasing metals," Michelle Jecmen, who is an undergraduate researcher at the University of Michigan and lead author of the study, said in a statement.
Related: James Webb Space Telescope sees major star factory near the Milky Way's black hole (image)
When the universe began, the Big Bang produced only the elements hydrogen and helium (with a smidgen of lithium). All the other elements came later, forged either within the bowels of stars or in the furnaces of their explosions. Astronomers call all those later elements "metals." These metals are now dispersed across the interstellar medium, finding their way into new star-forming regions and being incorporated into the next generation of stars. Although the details are not yet clear, the presence of specific metals within a star can subtly alter how that star evolves. For instance, scientists believe high-metallicity stars are more likely to produce a neutron star and a powerful supernova.
Get the Space.com Newsletter
Breaking space news, the latest updates on rocket launches, skywatching events and more!
Importantly, the blasts from multiple supernova explosions create a "wind" that can blow out any remaining molecular gas — gas that's fertile for forming stars.
More massive and more evolved galaxies, such as our Milky Way, have produced a higher abundance of metals over the eons during which they've churned through countless generations of stars. However, smaller dwarf galaxies have historically exhibited less star formation and therefore have more primitive compositions with fewer metals. But once a star-forming region in a dwarf galaxy does get going, it would appear that its stars' lower metallicities means they're more likely to produce black holes rather than powerful supernova explosions. Therefore, it likely takes longer for the region to become enriched with metals and start producing stars that go supernova with powerful winds that blow out all the gas.
"We argue that at low metallicity … there is a 10-million-year delay in the start of strong super-winds, which in turn results in higher star-formation," said Jecmen.
"Michelle's finding offers a very nice explanation," Jecmen’s supervisor and co-author of the study, Michigan astronomer Sally Oey, said in the statement. "These galaxies have trouble stopping their star formation because they didn’t blow away their gas."
Oey has led observations with the Hubble Space Telescope that found corroborating evidence for Jecmen’s model. Reporting on them in the Nov. 21 issue of The Astrophysical Journal Letters, Oey’s team targeted Markarian 71. Specifically, Oey was looking for triply ionized carbon. Atoms become ionized when they are struck by high-energy photons that can knock out an electron, leaving the atoms with a net positive charge. Triply ionized means an atom has lost three electrons.
The Hubble observations found an abundance of triply ionized carbon near the center of Markarian 71. Such triply-ionized carbon forms when gas is cooling and radiative outflows that are removing energy from the gas interact with warmer gas. But these cooling outflows should not exist if there was a hot super-wind blowing, such as with the winds from multiple supernovas, and those winds appear absent in Markarian 71.
The findings also provide insights into star-forming conditions in the early universe's first galaxies , realms that existed just a few hundred million years after the Big Bang. Galaxies during this period, which is referred to as "Cosmic Dawn" were also small, but with intense star formation and low metallicity. When observed, they often display evidence of gas clouds clumping together and ultraviolet light shining through the gaps between the clumps. Astronomers describe this as the "picket fence" model, like light from a setting sun shining through gaps in a garden fence.
A 10-million-year delay in the rise of supernova winds would explain why the gas in early galaxies has time to form such large clumps. "Looking at low-metallicity dwarf galaxies with lots of ultraviolet radiation is somewhat similar to looking all the way back to the cosmic dawn," said Jecmen.
It’s neat to consider that to learn things about the first galaxies, we don’t always need a $10 billion space telescope, but can rather just look at some of our diminutive neighbors.
A paper describing these findings was published on Nov. 21 in The Astrophysical Journal
Join our Space Forums to keep talking space on the latest missions, night sky and more! And if you have a news tip, correction or comment, let us know at: community@space.com.
Keith Cooper is a freelance science journalist and editor in the United Kingdom, and has a degree in physics and astrophysics from the University of Manchester. He's the author of "The Contact Paradox: Challenging Our Assumptions in the Search for Extraterrestrial Intelligence" (Bloomsbury Sigma, 2020) and has written articles on astronomy, space, physics and astrobiology for a multitude of magazines and websites.