'Modest, humble, and uncommonly smart': How a Soviet mathematician quietly solved the mystery of planet formation
"For years, he had the field of planetary formation, which he had created, virtually to himself. Most Soviet colleagues were skeptical and uninterested; his research appeared so speculative, so far removed from any evidence."
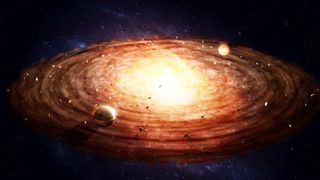
We've only got to grips with how the planets in our solar system formed in the last 100 years. In the extract below from "What's Gotten Into You" (HarperCollins, 2023), Dan Levitt looks at the Soviet mathematician who spent a decade working on a problem that most astronomers had given up on, and — when he finally solved it — was met with disinterest and skepticism.
Over 4.8 billion years ago, the atoms that would create us sailed in great clouds of gas and dust, toward… well, nothing. There was no solar system, no planets, no Earth. In fact, for a long time, scientists could not explain how our solid planet, not to mention one so hospitable to life, appeared at all. How was our now-rocky planet conjured, like magic, out of an ethereal cloud of gas and dust? How and when did Earth become so welcoming to life? And what travails were our molecules forced to brave until life could evolve?
Scientists would learn that our atoms could finally create life only after they endured wrenching collisions, meltdowns, and bombardments — catastrophes that beggar any destruction ever witnessed by humankind.
Explaining how our planets were created seemed so difficult that, by the 1950s, most astronomers had given up. Their theories appeared to lead nowhere. Two centuries before, the German philosopher Immanuel Kant and the French scholar Pierre-Simon Laplace had begun, promisingly enough, by correctly theorizing that gravity reeled in a massive spinning cloud of gas and dust so tightly that fierce temperatures and pressures ignited it into a star — our sun. But how did the planets form? They posited that a disk of stray dust and gases still remained spinning around the Sun, and this broke into smaller clouds that created the planets. However, no one could convincingly explain how the disk broke up or how the planets formed from these lesser clouds.
Related: This is our best look yet at how young stars stifle planetary formation
In 1917, the Englishman James Jeans took an inventive new tack that, as we saw, Cecilia Payne's contemporaries endorsed. Jeans surmised that the gravitational pull from a passing star was so strong that it wrenched massive chunks of gas from the sun's surface — and these became the planets. Others thought that our planets were debris left behind by the collisions of stars. But how nine distant planets formed from such a collision was anybody's guess. It seemed as likely as if you had put wet laundry in a dryer and then opened it to find your clothes not just dry, but neatly folded. Only a few astronomers continued to take the question seriously. It was a matter fit only for "innocent entertainment," or "outrageous speculation," observed the astronomer George Wetherill. It simply wasn't clear that we could ever see so far back in time.
Nevertheless, in the Soviet Union in the late 1950s, at the height of the Cold War, a young physicist decided to tackle the problem head on — with mathematics. His name was Viktor Safronov. Safronov was slight in stature and struggled with malaria, a legacy of his military training in Azerbaijan during World War II. He was modest, humble, and uncommonly smart. At Moscow University, he distinguished himself with advanced degrees in physics and mathematics. Recognizing his talent, the mathematician, geophysicist, and polar explorer Otto Schmidt recruited him to the Soviet Academy of Sciences.
Get the Space.com Newsletter
Breaking space news, the latest updates on rocket launches, skywatching events and more!
Schmidt himself, like Kant and Laplace before him, was sure that our planets had been created from a disk of gas and dust that orbited the Sun. He wanted someone with the technical skill to help him puzzle out how, and the soft-spoken Safronov was a brilliant mathematician.
In fact, his lack of a computer may have even helped, by forcing him to sharpen his already formidable intuition.
In an office at the Academy of Sciences, Safronov started at the beginning. He took upon himself the daunting task of trying to explain how trillions upon trillions of gas and dust particles could build a solar system. He would try to do it with mathematics — primarily statistics and equations of fluid dynamics, which describe the flow of gases and liquids. All this without computers. In fact, his lack of a computer may have even helped, by forcing him to sharpen his already formidable intuition.
Safronov began by assuming that our solar system first took shape when the vast primordial cloud of dust and gas, which in the previous chapter we left floating in space, was transformed by the relentless pull of gravity into a star. Almost all of it (99%, we know now) became our sun. But lingering remnants were too far away to be dragged into the sun, yet not distant enough to fully escape its clutches. Instead, gravity and the centripetal force of rotation flattened this cloud into a disk of dust and gases orbiting around the sun.
Safronov, who dazzled colleagues with his gift for making quick mathematical estimations, set out to compute what happened when tiny particles within the disk smacked into one another and then struck their neighbors. With pencil and paper and a slide rule, perhaps in the quiet of a library where Soviet scientists often retreated from the hubbub of large common offices, he doggedly attempted to estimate the effects of trillions upon trillions of collisions. That was an incredibly daunting endeavor, with or without a computer. By comparison, one would think that calculating the path of a hurricane from the initial water droplets forming in clouds would be child's play.
Safronov realized that the swarm of cosmic dust and gas orbiting the sun would be traveling around at roughly the same speed and direction. Sometimes, when the particles bumped into their neighbors, they would stick together like snowflakes. More collisions begat bigger and bigger clumps, until they were as large as boulders, ocean liners, mountain ranges, and eventually mini-planets. Building on his insight, Safronov single-handedly outlined most of the major problems scientists would need to solve in order to explain the origin of our planets. And with mathematical bravado, he conquered many of them.
For years, he had the field of planetary formation, which he had created, virtually to himself. Most Soviet colleagues were skeptical and uninterested; his research appeared so speculative, so far removed from any evidence. Then, in 1969, Safronov published a slim paperback, a retrospective of his decade of lonely work. He presented a copy to a visiting American graduate student, who passed it on to NASA with a recommendation that they have it published. Three years later, an English version appeared in the West.
It would revolutionize our understanding of how Earth and all planets are created.
Text from What's Gotten Into You: The Story of Your Body's Atoms, from the Big Bang Through Last Night's Dinner. Reprinted by permission of HarperCollins Publishers.
What's Gotten Into You: The Story of Your Body's Atoms, from the Big Bang Through Last Night's Dinner - $12.78 at Amazon
For readers of Bill Bryson, Neil deGrasse Tyson and Siddhartha Mukherjee, a wondrous, wildly ambitious, and vastly entertaining work of popular science that tells the awe-inspiring story of the elements that make up the human body, and how these building blocks of life travelled billions of miles and across billions of years to make us who we are.
Join our Space Forums to keep talking space on the latest missions, night sky and more! And if you have a news tip, correction or comment, let us know at: community@space.com.
Dan Levitt is the author of "What's Gotten Into You: The Story of Your Body's Atoms, from the Big Bang Through Last Night's Dinner." In the Peace Corps in Kenya, Dan taught high school physics, biology, and world history in a remote village. Living close to Mount Kilimanjaro, walking by anthills as tall as people, and seeing snakes, hippos, and other wildlife, gave him an intense curiosity about the natural world. He returned to Philadelphia to take a job developing exhibits and videos at the Franklin Institute Science Museum. That led to an interest in documentary filmmaking.
After getting an MFA, Dan moved to Boston and started his career writing, producing and directing documentaries for Discovery, Science, National Geographic, History, HHMI (Howard Hughes Medical Institute), and others. His work has received numerous awards including two Cine-Golden Eagles, Emmy award nominations, and the coveted Spur Award for script writing from the Western Writers of America.
While dreaming up films, Dan was seized by an idea for a book and decided to go for it. "What's Gotten Into You," published by HarperCollins is his first book.
-
rod It is interesting to look at trail blazers here and how the modeling for the solar nebula developed over the decades, what is presented today, and what is documented among the exoplanets now, example. https://skyandtelescope.org/astronomy-news/how-did-two-hot-and-super-dense-neptunes-form/Reply
My observation. Here is a 1977 report on the MMSN. The Distribution of Mass in the Planetary System and Solar Nebula, https://ui.adsabs.harvard.edu/abs/1977Ap%26SS..51..153W/abstract, September 1977. The arXiv paper, https://articles.adsabs.harvard.edu/pdf/1977Ap%26SS..51..153W, 19-Feb-1977.
The table in the paper shows MMSN values 0.01 to 0.07 solar masses and disk mass distribution for the planets so they can evolve from the disk. Total disk mass ranges 0.01 to 0.07 solar masses, 3.329428E+03 (3329.428) earth masses up to 2.330599E+04 (23305.99) earth masses in the protoplanetary disk said to evolve into the solar system we see today. The 1977, six-page paper at the end “4. Conclusions” indicates the MMSN creates an anomalously low mass region for Mercury, Mars, and the asteroids. Some values for the MMSN range 0.01 to 0.1 solar masses in the disk. 0.1 solar mass disk = 3.329428E+04 (33,294.28) earth masses. Much work is done now to refine the MMSN, dust, gas, and mass distribution in the postulated protoplanetary disk that creates the solar system we see today using a timescale of some 4.5 billion years or so. Comparing the 1977 MMSN to present day ALMA disk observations should be interesting too. Looks like much juggling takes place to get the protoplanetary disk to fall into place and create the solar system we see today. When I read the more current computer simulation models and reports like phys.org publishes or sometimes, space.com, I look for tables like the 1977 paper disclosing disk mass for different regions used in the simulations to create the solar system. Hard to find such clarity it seems.
My note. New computer models for simulating the origin of Mercury use perhaps 2 or 3 earth masses in the original, protoplanetary disk and still fail in many simulations. The terrestrial, rocky planets, Mercury, Venus, Earth, and Mars are difficult to show how they evolved from the postulated, early protoplanetary disk in our solar system. References, Dynamical Avenues for Mercury's Origin. I. The Lone Survivor of a Primordial Generation of Short-period Protoplanets, https://ui.adsabs.harvard.edu/abs/2021AJ....161..240C/abstract, May 2021. Dynamical Avenues for Mercury's Origin. II. In Situ Formation in the Inner Terrestrial Disk, https://ui.adsabs.harvard.edu/abs/2021AJ....162....3C/abstract, July 2021.
My note about the main belt asteroids in the MMSN. A typical orbital period is about 4.4 years so in one billon years, main belt asteroids could complete more than 227 million revolutions around the Sun in the heliocentric solar system. There is the potential for a wide range of primordial asteroid belt mass here (4.5 billion years old solar system model) because of the possible ejections and mass loss rate(s) compared to the present asteroid belt we see using telescopes. Then we have the exoplanet solar system TRAPPIST-1 with 7 exoplanets (about 5.6 earth masses total), all orbiting well inside of where Mercury is at in our solar system with computer simulations reporting the need for a large disk mass relative to the tiny, 0.08 solar mass host star in efforts to show accretion for these 7 exoplanets from the postulated primordial protoplanetary disk.