'Planet factories' may explain mysterious diversity of super-Earth alien worlds
The proposed formation process may be broadly applicable across solar systems.
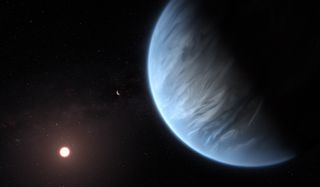
Super-Earths are the most common type of planet in the galaxy, but much remains unknown about how these mysterious worlds form.
Now, in a new study, researchers suggest they may be able to explain the origin of these enigmatic worlds and also that of other rocky planets and moons, including Earth and its siblings.
In the 30 or so years since astronomers first began detecting exoplanets orbiting distant stars, researchers have detected many strange new kinds of worlds unlike any known in our solar system. These include super-Earths, rocky worlds larger than our own at up to about 10 times Earth's mass. Some super-Earths possess atmospheres of hydrogen and helium, making them appear almost like gas giants.
Related: These 10 super extreme exoplanets are out of this world
Much remains uncertain about the underlying reasons for the differences between super-Earths and the solar system's terrestrial planets — Earth, Mercury, Venus and Mars. For example, super-Earths are typically much bigger than the solar system's terrestrial planets, with diameters approaching those of ice giants such as Uranus and Neptune. However, "recent data has revealed that extrasolar super-Earths are typically silicate-rich, much like the Earth itself," study lead author Konstantin Batygin, a planetary astrophysicist at the California Institute of Technology (Caltech) in Pasadena, told Space.com.
In addition, stars often host multiple super-Earths, and those revolving around a star together are often similar to each other in terms of size, mass, the spacing between their orbits and other key features. "Within a single planetary system, super-Earths are like 'peas in a pod,'" Andrew Howard, a professor of astronomy at Caltech who was not involved in the new study, said in a statement. "You basically have a planet factory that only knows how to make planets of one mass, and it just squirts them out one after the other."
However, although super-Earths usually prove similar to each other when they orbit the same star, there is a remarkable diversity of super-Earth types when many different stars are compared.
Get the Space.com Newsletter
Breaking space news, the latest updates on rocket launches, skywatching events and more!
"Adding to the difficulty of modeling super-Earth formation is the fact that our own solar system does not contain any super-Earths, except perhaps a very distant one," Batygin said.
Now researchers may have come up with a scenario that could explain not only the origin of super-Earths, but also that of the kind of rocky planets and moons that populate our own solar system. Batygin and his colleague Alessandro Morbidelli, a planetary scientist at the Côte d'Azur Observatory in Nice, France, detailed their findings online Thursday (Jan. 12) in the journal Nature Astronomy.
Previous research suggested planetary systems begin as large spinning disks of gas and dust. These "protoplanetary disks" consolidate over the course of a few million years or so, with most of the gas concentrating on the star at the center of the system, while solid material slowly coalesces into asteroids, comets, planets and moons.
To help explain how own solar system came to possess smaller rocky planets near the sun and larger gas giants farther away, in a 2021 study, Batygin and his colleagues suggested that planet formation in the solar protoplanetary disk involved two distinct rings — an inner one for the rocky planets and an outer one for more massive planets.
Now, in the new study, the scientists investigated whether this concept of multiple distinct rings in protoplanetary disks might help explain super-Earths as well.
"We looked at the existing model of planet formation, knowing that it does not reproduce what we see, and asked, 'What assertion are we taking for granted?'" Batygin said. "In this case, it turned out to be the commonly made assumption was that solid material within protoplanetary disks is uniformly dispersed. By jettisoning this assumption and instead supposing that the first solid bodies form in discrete rings, the new model can explain different types of planetary systems with a unified framework."
The researchers suggest that, during planetary formation, solid material concentrates in a narrow band in the protoplanetary disk where silicate vapors condense to form rocky pebbles.
"If you're a dust grain, you feel considerable headwind in the disk because the gas is orbiting a bit more slowly, and you spiral toward the star, but if you're in vapor form, you simply spiral outward, together with the gas in the expanding disk," Batygin said in a statement. "So that place where you turn from vapor into solids is where material accumulates."
Related: Solar system planets, order and formation: A guide
The scientists propose that this narrow band can act as a planet factory, with rocky worlds forming within it until they become large enough to exit the ring due to forces exerted by the gas. Over time, this process can produce several similarly sized rocky planets. This might help explain why all the super-Earths around one star might closely resemble each other but look quite different from the super-Earths around another star, which might have a significantly different planet factory.
If this ring contained a lot of mass, planets would grow until they migrate away, resulting in a planetary system containing similar super-Earths. If the ring contained less mass, it would produce worlds that look more like our solar system's terrestrial planets.
The researchers first came up with this idea of a narrow band giving rise to rocky bodies when they were exploring how to explain the formation of Jupiter's four largest moons — Io, Europa, Ganymede and Callisto. Galileo first spotted these satellites around the gas giant in the early 1600s.
"Over the years, it has been pointed out multiple times that extrasolar super-Earths look a lot like scaled-up variants of the Galilean moons," Batygin said. "But the physical nature of this connection had remained elusive. Among the most exciting aspects of our model is that it provides a theoretical framework that can explain the formation of the terrestrial planets of the solar system, extrasolar super-Earths, as well as the origins of the moons of Jupiter and Saturn, in a unified manner."
Follow us on Twitter @Spacedotcom or on Facebook.
Join our Space Forums to keep talking space on the latest missions, night sky and more! And if you have a news tip, correction or comment, let us know at: community@space.com.
Charles Q. Choi is a contributing writer for Space.com and Live Science. He covers all things human origins and astronomy as well as physics, animals and general science topics. Charles has a Master of Arts degree from the University of Missouri-Columbia, School of Journalism and a Bachelor of Arts degree from the University of South Florida. Charles has visited every continent on Earth, drinking rancid yak butter tea in Lhasa, snorkeling with sea lions in the Galapagos and even climbing an iceberg in Antarctica. Visit him at http://www.sciwriter.us
-
rod "Much remains uncertain about the underlying reasons for the differences between super-Earths and the solar system's terrestrial planets — Earth, Mercury, Venus and Mars. For example, super-Earths are typically much bigger than the solar system's terrestrial planets, with diameters approaching those of ice giants such as Uranus and Neptune. However, "recent data has revealed that extrasolar super-Earths are typically silicate-rich, much like the Earth itself," study lead author Konstantin Batygin, a planetary astrophysicist at the California Institute of Technology (Caltech) in Pasadena, told Space.com."Reply
The solar nebula and protoplanetary disc modeling runs into problems here as well as other areas of exoplanet studies (hot jupiters for example). Some of the origin theory modeling looks like efforts to avoid overturning the accepted paradigm.
Consider this report and discussion on exoplanets that are earth size, some of those are super-earth masses too.
https://forums.space.com/threads/james-webb-space-telescope-notches-1st-rocky-planet-confirmation.59557/
http://exoplanet.eu/, this site lists 371 exoplanets with masses 10 or less earths. Their radii range from 0.29 earth radii up to 20.18 earth radii size.
https://exoplanetarchive.ipac.caltech.edu/index.html, this site lists 450 exoplanets in the 10 or less earth mass range. Radii range 0.3 earth radii to 36.37 earth radii size. Such exoplanet finds challenge the solar nebula model answers as to how Mercury, Venus, Earth, and Mars formed with their smaller masses and diameters.
Comments like this in the article are important to keep in mind. "Much remains uncertain about the underlying reasons for the differences between super-Earths and the solar system's terrestrial planets — Earth, Mercury, Venus and Mars." -
rod Here is another report on super-earths by space.com.Reply
Alien super-Earths may get a habitability boost from hydrogen-rich atmospheres, https://forums.space.com/threads/alien-super-earths-may-get-a-habitability-boost-from-hydrogen-rich-atmospheres.56415/
"...In the new study, researchers investigated whether a super-Earth enveloped in these gases might prove habitable. If the atmosphere of such a world is massive enough, its hydrogen can serve as a greenhouse gas, trapping heat from the star despite the planet's distance, the scientists noted. The scientists developed computer simulations of super-Earths between one and 10 times Earth's mass with atmospheres rich in hydrogen and helium that orbited sun-like stars at distances of 1 to 100 astronomical units (AUs). (One AU is the average distance between Earth and the sun, which is about 93 million miles, or 150 million kilometers.) The researchers found that super-Earths at distances greater than 2 AU could host temperate conditions and liquid water on their surfaces for up to somewhere between 5 billion and 8 billion years. Such findings suggest "we should also consider exotic habitats when we are investigating habitability on other planets," Mol Lous said. These kinds of super-Earths could prove habitable even if they go "rogue" — that is, wander through space not bound to a star. Recent work has suggested that free-roaming planets may be common."
Plenty of modeling assumptions needed for super-earths. -
rod "Now researchers may have come up with a scenario that could explain not only the origin of super-Earths, but also that of the kind of rocky planets and moons that populate our own solar system. Batygin and his colleague Alessandro Morbidelli, a planetary scientist at the Côte d'Azur Observatory in Nice, France, detailed their findings(opens in new tab) online Thursday (Jan. 12) in the journal Nature Astronomy."Reply
I found this report and 14-page PDF that may be easier to read through :)
Formation of Rocky Super-Earths From A Narrow Ring of Planetesimals, https://arxiv.org/abs/2301.04680
My note. This is a key concept concerning the model to explain the origin and formation of super-earths found and no super-earths observed in our solar system. “Our results thus indicate that the absence of short-period super-Earths within the solar system can simply be attributed to the comparatively low mass of the primordial planetesimal ring within the protosolar nebula.”
My note. A 3 Myr period used in the simulations of protoplanetary discs to create super-earths stated in the paper. “Cumulatively, we carried out our calculations over a timespan of 3 Myr, in agreement with typical lifetimes of protoplanetary disks.”
My note, the growth ring used in the simulation is 20 earth masses as reported. “The initial embryo masses used in our simulations were informed by particle-in-a-box calculations of collisional growth within a 20Mearth planetesimal ring.” My note. The 1977 MMSN model used 639 earth masses from 0.22 au to 2.0 au to explain the origin of Mercury to Mars, and asteroids. This super-earth model uses 20 earth masses in the postulated ring in the protoplanetary disc to show super-earth creation. As stated in the PDF, a lower amount of mass for our solar system from Mercury to the asteroid belt in a ring(s) is needed so no super-earths form in our solar system apparently. When I read about new models developed to explain the origin of exoplanets and our solar system, I go back and compare to the 1977 model that I use as a baseline for comparison.
The Distribution of Mass in the Planetary System and Solar Nebula, https://ui.adsabs.harvard.edu/abs/1977Ap%26SS..51..153W/abstract -
Dragrath Regarding the explanations behind the diversity of exoplanets its also probably important to account for the innate biases in our assumptions and models for planet formation we also have to factor in planet evolution as well since there is pretty strong evidence that the conditions on Venus, Earth, Mars and even the orbital dynamics of Saturn have changed considerably since they initially formed, in fact all the planets as we know them are still dynamically changing thus was we see around older stars may not be representative of their formation conditions.Reply
There was an interesting paper Anton Petrov brought to attention some years back with looked at the Kepler catalog and looked at the distribution of planets in size and compared that with their estimated age. While still obviously quite crude one standout result was that younger stars (those less than a billion years old) had larger diameter planets particularly an overabundance of "Mini Neptunes" whereas older stars (those more than a billion years old) show a higher abundance of "super Earths". The paper concluded that atmospheric escape is probably the best explanation for the difference.
This if verified by future work may also explain some of the extremely dense massive close in planets around old M dwarfs since if a gaseous world was evaporated its compressed rocky core might have become crystalized in such a form compensating for lower astrophysical metal abundances of their host stars.
From the perspective of geology however is where I come to support this kind of model most because as we have developed seismic tomography to probe the Earth's interior structure and began better characterizing magma chemical compositions and temperatures it has become apparent that there is significant thermochemical activity occurring deep inside our planet which interestingly has some surprisingly strong temporal cross matching to major ecological and climatic shifts in our planets history.
https://www.nature.com/articles/s41598-019-43103-y#citeas
In particular the temperature dependence of the phase transition from silicate rocks to hydrated silicates appears to be inversely proportional meaning that the reaction is inhibited in hot conditions probably in part because such processes are exothermic releasing heat thus the hotter Earth's mantle the less mineral hydrates can exist thus the more water will be able to exist in liquid form. This may help explain some curious observations related to the Early Earth namely the rate at which Earth's oceans formed, the lack of chemical isotopic signatures of atmospheric rock geochemistry prior to 3 billion years ago(Ga), and the peculiar observation based on geophysical evidence such as paleomagnetism fossils mineral types with time that the rate of motion and depths involved in plate tectonics on Earth have increased over time rather than decreased as would be expected were it purely fueled by thermal processes.
The early oceans can be explained if all that water was once in the oceans since given the sheer quantity it could have naturally allowed the water vapor in the aftermath of the Proto-earth Theia collision to have high enough temperatures and pressures to become supercritical fluids. This would mean there is no need for condensation type phase transitions since supercritical fluids don't undergo hard phase transitions able to naturally relax into a mix of liquid and gas phases in relatively equal proportions. In this case you could naturally have supercritical water oceans form on top of the still molten Earth before the crust even begins to solidify. This isn't far fetched as in the deep sea around hydrothermal vents supercritical water naturally exists on modern Earth so its existence on a hotter deeper ocean world is kind of trivial in this sense water would have behaved more like it does in Uranus and Neptune than the familiar surface water, plus the interface between a roiling molten magma ocean and a supercritical water ocean could be a place where exotic chemistry can potentially occur such as the reaction between iron and nickel rich volcanic glass(which is a solid which has frozen into the chemical structure of a liquid) which has been shown to readily catalyze the formation of RNA. could some more extreme chemical analog have seeded life on Earth?
In fact there is likely a deeper biological connection to this cycle as the 3 geophysical states of plate tectonics which we find in the rock record also correspond roughly to the Paleozoic + late Neoproterozic with "modern" plate tectonics and high levels of atmospheric oxygen, the Mesoproterozoic and Paleoproterozoic with a dynamic yet slower more shallow/less vigorous kind of tectonics and small percentages of oxygen in the atmosphere or shallow environments but otherwise completely anaerobic world often called the "boring billion" and then the old Archean eon and older which seem to have behaved even more unusually and for which there is evidence that while the continents physically existed to some degree they were likely fully submerged.
The following paper using modern analogs of microbial metabolisms explains an ecological reason for why these 3 different epochs of life each of which appears to have been divided by extreme and rapid transitions between a "snowball" and hot house state.
https://www.nature.com/articles/s41467-019-10872-z#citeas
Effectively aerobic carbon fixation is ecologically limited by competition since it requires more energy to use water as a source of molecular hydrogen for carbon fixation. Thus in a water world where upwards passive diffusion is the primary source of mineral nutrients other methods of obtaining hydrogen for carbon fixing aerobic primary production is ecologically nonviable as nutrients will be exhausted by other anaerobic forms of photosynthesis which can use less energetic light before they can rise to the depths at which aerobic photosynthesis (which either requires at least 3 "red" photons or 1 "blue" to successfully split water into oxygen and molecular hydrogen) is possible.
This comes down to oxygen having the second highest electronegativity of all chemical elements that are not noble gasses and thus is the flip side of what makes aerobic respiration so efficient.
As a result aerobic carbon fixation requires too much energy for chemical processes or longer wavelengths of light (particularly infrared light) which are targeted by other kinds of anaerobic photosynthesis thus only in very shallow waters where sunlight can reach the bottom(or where some process saturates the water with abundant nutrients to such a degree that they are no longer a growth limiting factor) can aerobic photosynthesis become ecologically viable.
The aforementioned mantle hydration threshold happens to line up suspiciously well with the timing when such shallow water conditions would have been possible assuming all of Earth's water was initially in our oceans and has been subsequently sequestered away through crustal and mantle hydration processes. And that happens to line up within a few hundred million years or less of the Great Oxygenation Event(GOE).
Now as for why oxygen levels plateaued soon after the GOE, it turns out that while models have long assumed that anaerobic organisms can't stand oxygen that is only partly true since it has to do with oxygen saturation levels there turns out to be a lot of modern bacteria primarily in the soil and midwater depths which not only engage in anaerobic primary production/carbon fixation but use aerobic respiration primarily or even in some cases exclusively.
From what I have read these bacterial groups are actually extremely abundant at least in pelagic environments where they account for at least 20% to the total bacterial biomass in Earth's oceans. Its hard to know exactly how abundant they are because unfortunately like most microorganisms aside from laboratory favorites which have been studied to death microorganisms have been and continue to be deeply understudied with many likely having yet to be discovered by modern science since we are still discovering entirely new forms of metabolism and their associated ecological niches and roles which we had no idea even existed all the time in the prokaryotic microcosmos. Some of these have turned out to be major essential roles in the cycling of essential mineral and elemental nutrients particularly in regards to decomposition so we aren't talking about obscure minor players but critical parts of Earth's biosphere of which there absence would have catastrophic consequences as certain waste products would accumulate to dangerous levels.
The two largest bacterial groups which appear to have long specialized in this anaerobic photosynthetic/photoreactive metabolism with facultative and or obligate aerobic metabolisms strategy are the alphaproteobacteria and gammaproteobacteria. Of these notably the alphaproteobacteria are the clade from which mitochondria descend hence they are pretty important all things considered.
Some more potential evidence for this kind of distinction comes from metagenomic sampling which lets us sequence things in an environment without having to culture them in the lab, (which turns out to miss the vast majority of microbes because only a small percentage can actually consume the bovine serum they tend to use to culture microbes), as this has revealed a surprising link between Eukaryotes and particular thus far uncultured Asgard Archaea lineages thus far isolated only from continental environments (soil or freshwater sediments) with aerobic microbes in marine environments turning out to have diverged from continental kin only during the Neoproterozoic (meaning the open oceans were as mineral studies have suggested, likely fully anaerobic up until around ~800 million years ago or so)
In fact given the studies of cyanobacteria Eukaryogenesis was likely restricted to freshwater basins since the cyanobacteria ancestor of chloroplasts is part of a lineage which is obligate freshwater (low salinity) organisms. All together it suggests complex life arose within the continental waters and only far later after over a billion years of adaptation began to colonize the open oceans.
This is where the newest and most familiar tectonic regime comes into play as hydrous mantle plumes turn out to need a sufficiently high saturation of hydrated rocks to accumulate above subducted slabs these magmas which are rich in elements derived from subducted sediments most notably phosphorus thus became able to rise back to the surface in chemically derived mantle plumes. It is at this point that the modern "deep" subduction appears to have begun with the vigorous break up of Rodinia and a profusion of subduction activity as magmas related to supercontinent break up carry the chemical fingerprints of sediment enhanced chemically buoyant mantle.
TDLR the physical evolution of planets may not only be instrumental in shaping planets but we probably owe our existence to it. -
rod Post #5 commented, "Regarding the explanations behind the diversity of exoplanets its also probably important to account for the innate biases in our assumptions and models for planet formation we also have to factor in planet evolution as well since there is pretty strong evidence that the conditions on Venus, Earth, Mars and even the orbital dynamics of Saturn have changed considerably since they initially formed, in fact all the planets as we know them are still dynamically changing thus was we see around older stars may not be representative of their formation conditions."Reply
Some interesting thinking here. However, when it comes to the origin of our solar system and comparing with exoplanets documented today, there are apparent biases. Just look at the MMSN in the protoplanetary disc modeling used for our solar system as an example, the dust and gas mass variations used in simulations. If Venus, Earth, Mars orbital dynamics changed, that should be clearly shown to the public. There are initial positions, conditions, and where they are at today relative to the Sun, including initial masses and radii, including what time period is used for simulations and planet migration scenarios invoked. The zoo of exoplanets is more than challenging to fit within the MMSN model used for our solar system. So is explaining how Venus and Earth evolved in the MMSN and disc model - so obviously very differently with vastly different outcomes, all within the Sun's habitable zone.
In post #2 I listed two very good exoplanet sites I used in my personal studies. The exoplanet.eu site lists 355 with masses between 1.1 and 10.5 earth masses. The NASA archive site lists 443. The average mass at the .eu site is 5.62 earths, at the NASA site, 5.8 earth masses. Distances from host stars range 0.006 to 32 au for the exoplanet.eu site, NASA site, 0.00716 to 10.26 au and star masses range 0.06 solar mass to 2.24 solar mass. The majority of exoplanets reported at these sites do not report the mass, the majority listed do show their radii sizes. It is not hard to compare here using the confirmed exoplanets with our solar system, thus *innate biases in our assumptions* at least to me, stand out sharply when I review this subject. -
rod https://forums.space.com/threads/nasa-scientist-explains-why-venus-is-earths-evil-twin-video.59691/Reply
Some interesting info on Venus and Earth at the link. -
Dragrath
Well the lack of masses for most planets tells me they were discovered via the transit method and follow up radial velocity analysis is either not possible at current precision or has not yet been done.rod said:Post #5 commented, "Regarding the explanations behind the diversity of exoplanets its also probably important to account for the innate biases in our assumptions and models for planet formation we also have to factor in planet evolution as well since there is pretty strong evidence that the conditions on Venus, Earth, Mars and even the orbital dynamics of Saturn have changed considerably since they initially formed, in fact all the planets as we know them are still dynamically changing thus was we see around older stars may not be representative of their formation conditions."
Some interesting thinking here. However, when it comes to the origin of our solar system and comparing with exoplanets documented today, there are apparent biases. Just look at the MMSN in the protoplanetary disc modeling used for our solar system as an example, the dust and gas mass variations used in simulations. If Venus, Earth, Mars orbital dynamics changed, that should be clearly shown to the public. There are initial positions, conditions, and where they are at today relative to the Sun, including initial masses and radii, including what time period is used for simulations and planet migration scenarios invoked. The zoo of exoplanets is more than challenging to fit within the MMSN model used for our solar system. So is explaining how Venus and Earth evolved in the MMSN and disc model - so obviously very differently with vastly different outcomes, all within the Sun's habitable zone.
In post #2 I listed two very good exoplanet sites I used in my personal studies. The exoplanet.eu site lists 355 with masses between 1.1 and 10.5 earth masses. The NASA archive site lists 443. The average mass at the .eu site is 5.62 earths, at the NASA site, 5.8 earth masses. Distances from host stars range 0.006 to 32 au for the exoplanet.eu site, NASA site, 0.00716 to 10.26 au and star masses range 0.06 solar mass to 2.24 solar mass. The majority of exoplanets reported at these sites do not report the mass, the majority listed do show their radii sizes. It is not hard to compare here using the confirmed exoplanets with our solar system, thus *innate biases in our assumptions* at least to me, stand out sharply when I review this subject.
In the context of initial simulations yeah there are huge biases probably the most glaring of them is that observations of young systems have been showing that planetary systems can form much more quickly than primarily accretionary models can reproduce. Instead evidence appears to be mounting for another type of model known as disk instability driven direct collapse. Current evidence for this includes the object 486958 Arrokoth which appears to preserve a fossil of this kind of initial collapse, the internal structure results of Jupiter Saturn and Mars indicating that these 3 planets are much less differentiated than we expected based on the study of Earth and our Moon (Gee its almost as if Earth and the Moon as we know them may have formed together in a different mechanism or something like two planets smashing into each other and getting vaporized/liquified in a dynamical mess). There is also some isotopic analysis that have been done on Earth's nitrogen and water and thus far they are both consistent with the isotopic ratios of undifferentiated stony chondritic material rather than the icy isotopic ratios richer in heavier isotopes.
And in the case of water the total amount of water to rock also matches what would be expected for this material thus it suggests that the planets likely formed quickly such that there was little opportunity for lighter isotopes to escape before differentiation could lead to loss of lighter gases as would be expected from small planetesimal accretion.
In this context disk instability of say astrophysical dust grains within the molecular region of an accretion disk or if further out dust and icy grains could clump up under the chaotic turbulent conditions with more massive clumps being potentially able to form directly from large clumps of gas to form giant planet or stellar massed objects.
This might also help solve another mystery related to how accreting bodies can grow so quickly as some recent work studying protostars has found energetic outbursts where it appears that accretion around the baby star is occurring in bursts of several Jupiter masses rather than a steady rate. Moreover there are some interesting examples of accretion elsewhere such as high mass protostars with stellar mass objects imbedded within their accretion disks or the S objects around SagA* appearing to be primarily aligned in a planar disk and containing many very massive stars which should only last a few million years before the burn out of fuel. (And potentially less related but still worth noting is how recent work has shown that the black hole mergers observed via gravitational waves appear to have fairly significant kickback/recoils capable of expelling such objects from globular cluster like environments which makes conventional compound mergers difficult however what about massive bodies imbedded within an accretion disk? If the sort of stars we see around Sag A* which appear to likely be products of its past accretion exist then it goes to suspect that they might be able to burn through their fuel go supernovae and continue accreting as compact stellar remnants eventually becoming black holes which slowly in fall within the accretion disk until they either collide with each other and or collide with the SMBH at the center thus bypassing accretionary limits. -
rod Dragrath in post #8 said, "Well the lack of masses for most planets tells me they were discovered via the transit method and follow up radial velocity analysis is either not possible at current precision or has not yet been done."Reply
Correct. The exoplanet.eu site shows 5306 confirmed, primary transit used for 3709. Radial velocity 1028. Similar for the NASA archive site too (5243 confirmed), this site indicates 91% transit method. What does this suggest? Real properties for many exoplanets are not well known, including atmospheres.
http://research.iac.es/proyecto/exoatmospheres/index.php, this site shows 144 with atmospheres and molecules determined, some show featureless spectra.
When I looked at the exoplanet.eu site for exoplanets with semi-major axis 3 or less AU from their stars (like main belt asteroids here to Mercury or closer in our solar system) and radii published, 3204 found and 2236 showed radii ranging 0.01 earth radii up to 8 Jupiter radii within that distance from their parent stars. The NASA site not much different. 4650 reported with min earth radii 0.3 up to 2.76 Jupiter radii size. There are plenty of large exoplanets documented now orbiting within the area of their host stars where in our solar system we have Mercury out to main belt asteroids. A very different configuration in our solar system compared to many exoplanets reported now. -
Helio
Interesting, so I thought I would see what the current data shows....Dragrath said:There was an interesting paper Anton Petrov brought to attention some years back with looked at the Kepler catalog and looked at the distribution of planets in size and compared that with their estimated age. While still obviously quite crude one standout result was that younger stars (those less than a billion years old) had larger diameter planets particularly an overabundance of "Mini Neptunes" whereas older stars (those more than a billion years old) show a higher abundance of "super Earths". The paper concluded that atmospheric escape is probably the best explanation for the difference.
It's a bit crude since I didn't bin the data, but perhaps it will serve us just as well.
From the perspective of geology however is where I come to support this kind of model most because as we have developed seismic tomography to probe the Earth's interior structure and began better characterizing magma chemical compositions and temperatures it has become apparent that there is significant thermochemical activity occurring deep inside our planet which interestingly has some surprisingly strong temporal cross matching to major ecological and climatic shifts in our planets history.
That's interesting. How much of the interior heat comes from thermochemical reactions? Convection and radioactive decay, along with the initial formation heat, has been all that I've seen for the heat flux from the interior.
... which has been shown to readily catalyze the formation of RNA. could some more extreme chemical analog have seeded life on Earth?
This seems to favor the ocean vent model for proto-life on Earth. Interesting.
Effectively aerobic carbon fixation is ecologically limited by competition since it requires more energy to use water as a source of molecular hydrogen for carbon fixation. Thus in a water world where upwards passive diffusion is the primary source of mineral nutrients other methods of obtaining hydrogen for carbon fixing aerobic primary production is ecologically nonviable as nutrients will be exhausted by other anaerobic forms of photosynthesis which can use less energetic light before they can rise to the depths at which aerobic photosynthesis (which either requires at least 3 "red" photons or 1 "blue" to successfully split water into oxygen and molecular hydrogen) is possible.
Photosynthesis? Lucky us. :) Ironically, the red dwarfs are the ones weak in these photons.
I see you did subsequently state "aerobic photosynthesis".
Your post is very powerful and deserves some attention, but its depth requires scuba gear for me and, likely, others. I wish I could convert your presentation to some key points, but if it's possible to do so, please do so. -
Helio The OP uses "super Earths" to mean anything between 1 and 10 Earth masses. There are 1826 exoplanet masses in the database (exoplanet.eu), and 3851 that state radii. There doesn't seem to be an established classification system yet, perhaps for good reason till more is understood. Or, I perhaps I'm wrong.Reply
Sky & Telescope had an article (Feb 2021) that used classes, so the following is based on those: