How did supermassive black holes get so big so fast just after the Big Bang?
"It's like seeing a family walking down the street, and they have two six-foot teenagers, but they also have with them a six-foot-tall toddler."
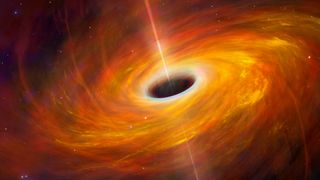
Scientists now understand that supermassive black holes lurk at the heart of most, if not all, galaxies. These cosmic titans have masses millions and even billions of times that of the sun, yet tremendous size doesn't constitute a problem when supermassive black holes are seen in the local universe and thus more recent in cosmic history.
Supermassive black holes become an issue, however, when they are seen in the early universe, and they already have masses equivalent to billions of suns. That is because there must be some mechanism that allows supermassive black holes to rapidly gather mass and grow to such giant sizes, yet all the existing mechanisms for this growth suggest this process proceeds too slowly for objects like this to exist just after the Big Bang.
"Over the last two decades, astronomers have found supermassive black holes with the same masses as in the local and thus more recent universe — billions of solar masses — almost 13 billion years ago, less than a billion years after the Big Bang," Royal Society University Research Fellow at Maynooth University John Reagan told Space.com.
Regan describes the problem with a somewhat disturbing analogy. "It's like seeing a family walking down the street, and they have two six-foot teenagers, but they also have with them a six-foot tall toddler. That's a bit of a problem, how did the toddler get so tall? And it's the same for supermassive black holes in the universe. How did they get so massive so quickly?"
Related: What is a black hole event horizon (and what happens there)?
This issue was further complicated this year when the James Webb Space Telescope (JWST) discovered the most distant and earliest supermassive black hole. Located at the heart of the galaxy CEERS 1019, this black hole has a mass of 9 million times that of the sun, which makes it relatively diminutive for a supermassive black hole.
Yet, even at this size, the existence of this just 570 million years after the Big Bang is challenging to theories of black hole growth. And this 9 million solar mass black hole wasn't alone. The same observing campaign that revealed this supermassive black hole, the Cosmic Evolution Early Release Science (CEERS) Survey, also uncovered two other supermassive black holes that existed both 1 billion years and 1.1 billion years after the Big Bang.
"With each new discovery, the constraints on our existing ideas become stronger," Canadian University of Western Ontario professor Shantanu Basu told Space.com. "We were worried when supermassive black holes were seen 800 million years after the Big Bang. CEERS just increases the challenge tremendously."
This suggests that supermassive black holes are common in the relative infancy of the universe, not some cosmic rarity, thus putting more pressure on the search for a mechanism to explain how they got there.
How do black holes control their own diets?
Discounting primordial black holes hypothesized to be left over after the Big Bang, the three main categories of black holes are stellar mass black holes, which have mass between 5 and 100 times the sun, intermediate-mass black holes with masses between 100 and 10,000 times that of the sun, and supermassive black holes with the masses mentioned above.
Stellar-mass black holes form when the most massive stars, with between 30 to 130 solar masses, run out of fuel for nuclear fusion and can no longer support themselves against their own gravity. As the outer layers of these stars are blown away in huge supernova explosions, the cores collapse to create stellar-mass black holes–regions of space with a point of infinite density called a singularity at its center and an outer boundary called the event horizon where the force of gravity is so great not even light can escape it.
Supermassive black holes must form in a different way from stellar mass black holes, as it is impossible for a star to be large enough to have the initial starting mass to shed mass as it evolves through events like the supernova that accompanies the gravitational collapse of the star but still leave behind a core massive enough to become a supermassive black hole.
For many years astronomers have believed that supermassive black holes could start their lives as "seed black holes" that are much smaller. Growing by first feeding on material and then by merging with other black holes when the galaxies they reside in collide, something that also provides these budding supermassive black holes with gas and dust to feed on.
The growth of stellar-mass black hole seeds could happen when these cosmic seeds find themselves surrounded by vast amounts of matter and greedily feast on this material to rapidly grow into supermassive black holes.
That process should be stunted, however, by something called the Eddington limit. The luminosity or brightness of a feeding black hole is proportional to the rate at which they are gathering mass. The faster a black hole consumes matter, the faster it grows, and the faster it grows, the more electromagnetic radiation is blasted out by its surroundings. But, if the electromagnetic radiation emitted from around a black hole in the form of jets is intense enough, it physically pushes material away. This means the faster a black hole "eats," the more likely it is to have its food supply interrupted and pushed away, thus halting that growth.
The Edington limit means that it should take black holes billions of years to accrete enough matter to reach supermassive black hole status. Regan is part of a team of researchers that investigated something called "super Eddington accretion," which could account for the rapid growth of supermassive black holes in the early universe. This wouldn't, he explained, be anything particularly special, just a more rapid version of normal black hole feeding
This would lead to rapid "episodes" of feeding with material pushed away by jets halting feeding and thus cutting off the jets. This then allows the material to fall back to the black hole, thus triggering another bout of frenzied feasting. Regan and colleagues found this explanation unsatisfactory, however.
"If you average this feeding cycle over time, it is actually less than the Eddington rate," Regan said. "It might be okay for one or two cycles, but overall, over time, it's not great because it just can't it doesn't go continuously. So we didn't find that this was not a great mechanism to really explain supermassive black hole growth."
Related: How does astronomy use the electromagnetic spectrum?
Can black holes take a shortcut to supermassive status?
Another possibility that could help explain the rapid growth of supermassive black holes is the idea that the seed black hole from which they grow is massive in size, according to Regan.
"We have light seeds and heavy seeds, so supermassive black holes could be born at 100 solar masses and grow all the way to supermassive black holes, or they could start off 100,000 more massive than the sun and grow from there," Regan said. "If they want to grow, a small black hole has to get exceptionally lucky and find itself in a dense environment where there's lots and lots of gas around. But that's very unlikely.
"It's much, much, much more likely than a little black hole to find itself in an environment with no gas. So it's very unlikely those small black hole seeds can grow."
Massive black hole seeds would still have to get into these dense environments, but at least they would have a head start on smaller seeds. Going back to the six-foot infant analogy, Regan explains that the growth of this child is even tougher to explain if it was born with the length of an average infant. But, if this baby was born and it was already three feet long, its transformation to a six-foot-tall toddler isn't quite as tough to explain.
The problem with investigating this is, unlike toddlers, black holes lack any characteristics beyond their mass, angular momentum (spin) and their electric charge. Scientists call this the "no-hair theorem," and it means that black holes are deceptively simple and don't carry information about their histories or evolution.
"If you find a black hole in your garden, you can't know by looking at it, don't know if it arrived there yesterday, a minute ago, or a billion years ago. It has no history, there's no fingerprint," he continued. "So when we look at black holes either today or 13 billion years ago, We have no idea what its age is, so we don't know how long it has been able to grow for."
Basu added that observing the feeding process that is bulking up black holes in the early universe hasn't been possible as it is happening so far away from us, but detecting supermassive black hole feeding may be possible in the future. "The hypothetical supermassive stars in the early universe would be extremely luminous, perhaps ten billion times as bright as our sun. It's possible that the James Webb Space Telescope (JWST) or Euclid could detect such objects if they are present as late as a few hundred million years after the Big Bang."
Despite these issues, Regan is confident that by investigating black holes in the early universe and by potentially discovering heavy seeds, scientists will soon be able to build a supermassive black hole growth picture. In particular, he pointed to the launch of the Laser Interferometer Space Based Antenna (LISA), a space-based gravitational wave detector that will help scientists better constrain the demographics of black holes in the early universe.
"I think we made a huge amount of progress in the last 10 years. Huge. And we will continue to make massive progress over the next decade as well.," Regan said." Extrapolating out from these demographics will give us a very, very good handle on the number of mergers happening in the distant universe involving black holes in exactly the mass range we need, with masses around 100,000 times that of the sun.
"I think it is very probable we will have solved this problem in the next 5 to 10 years."
Additional resources
You can read more about the James Webb Space Telescope's pioneering CEERS survey which is discovering black holes in the early universe here: https://ceers.github.io/
Bibliography
J. A. Regan., T. P. Downes., M. Volonteri., et al, Super-Eddington Accretion and Feedback from the First Massive Seed Black Holes, [2018], [https://arxiv.org/abs/1811.04953]
Webb Telescope Detects Most Distant Active Supermassive Black Hole, UT News, [2023], [https://news.utexas.edu/2023/07/06/webb-telescope-detects-most-distant-active-supermassive-black-hole/]
C-H. Lin., K-J Chen., C-Y. Hwang., Rapid Growth of Galactic Supermassive Black Holes through Accreting Giant Molecular Clouds during Major Mergers of Their Host Galaxies, [2023], [https://iopscience.iop.org/article/10.3847/1538-4357/acd841]
S. Batu., A. Das., The Mass Function of Supermassive Black Holes in the Direct-collapse Scenario, [2019], [https://iopscience.iop.org/article/10.3847/2041-8213/ab2646]
Join our Space Forums to keep talking space on the latest missions, night sky and more! And if you have a news tip, correction or comment, let us know at: community@space.com.
Get the Space.com Newsletter
Breaking space news, the latest updates on rocket launches, skywatching events and more!
Robert Lea is a science journalist in the U.K. whose articles have been published in Physics World, New Scientist, Astronomy Magazine, All About Space, Newsweek and ZME Science. He also writes about science communication for Elsevier and the European Journal of Physics. Rob holds a bachelor of science degree in physics and astronomy from the U.K.’s Open University. Follow him on Twitter @sciencef1rst.
-
rod "Despite these issues, Regan is confident that by investigating black holes in the early universe and by potentially discovering heavy seeds, scientists will soon be able to build a supermassive black hole growth picture. In particular, he pointed to the launch of the Laser Interferometer Space Based Antenna (LISA), a space-based gravitational wave detector that will help scientists better constrain the demographics of black holes in the early universe. "I think we made a huge amount of progress in the last 10 years. Huge. And we will continue to make massive progress over the next decade as well.," Regan said." Extrapolating out from these demographics will give us a very, very good handle on the number of mergers happening in the distant universe involving black holes in exactly the mass range we need, with masses around 100,000 times that of the sun. "I think it is very probable we will have solved this problem in the next 5 to 10 years."Reply
My observation. I am not so sure about this, *I think we made a huge amount of progress* concerning the origin of various SMBH documented now. Space.com reports recently also show Population III stars as possible seeds, some 1,000 to 10,000 solar masses or more. Other reports on inflation, in the post-inflation epoch can create a plethora of PBH, primordial black holes that could fill the universe today, and if they grew over postulated billions of years after the postulated BB event, could be very big today :) -
rod https://forums.space.com/threads/supermassive-black-holes-grow-surprisingly-quickly-study-suggests.62025/Reply -
starnut If the idea that all of everything was squeezed into a something the size of a grapefruit that exploded, I will not be surprised to find out that after the big bang some of the pieces of the grapefruit stayed in their original form to some extent. Assuming this is even possible.Reply -
Harmonograms The section describing Black Hole Diet is illogical and doesn't conform to BH observations; particularly this quote; "But, if the electromagnetic radiation emitted from around a black hole in the form of jets is intense enough, it physically pushes material away." The relativistic jets are pointed north and south and at right angles to the material entering the BH by way of the accretion disc, the jets are NOT interfering with the material entering by way of the accretion disc vector. What is actually shutting off the BH's feed is the loss of gravity the relativistic jets represent, because they also carry off vast amounts of mass (one estimate says 40% of incoming mass may be ejected) from the vicinity of the BH and spew it into intergalactic space. This loss of mass and gravity from the space around the BH lessens it's overall gravitational attraction which stabilizes the orbit's of debris which would otherwise auger into the BH. The jets shut off the BH's feed by lowering the overall mass and gravity around the BH, not by directly pushing the mass away (which is not observed). This is why BHs tend to become less active as they age.Reply -
Unclear Engineer While the jets are not "pushing" on matter in the accretion disk. aren't they electromagnetically "pulling" matter from the accretion disk into the jets?Reply -
Harmonograms
I'm sure the intense electromagnetic currents have an important effect on the distribution of mass within the accretion disc and the relativistic jets. It has been observed that the jets revolve in the same direction as the accretion disc and I believe that's an important clue. I wish we could see how this all works and what's going on within the event horizon.Unclear Engineer said:While the jets are not "pushing" on matter in the accretion disk. aren't they electromagnetically "pulling" matter from the accretion disk into the jets? -
billslugg The black hole accretion disc is charged particles, half of them positive and half negative. Heavy positive protons are less mobile than light negative electrons so there is a net electrical current running in a circle around the equator. This produces a bipolar magnetic field. Gravity and angular momentum draw gasses into closer, faster orbits generating shear forces and heat. Gas wants to escape but can't because, except at the poles, the charged particles would have to cross magnetic field lines to escape. very hard to do. Much easier to escape at the poles.Reply -
Atlan0001 Electroweak force (EWF) magnetic monopole point singularities upscaled into the macro-verse as black holes are vastly materially harder than either neutron stars or magnetars because -- as I for one visualize them now -- they are sheer 100% force fields, and nothing but magnetic monopole singularity, through and through and the strongest magnetic force, as well as superconductor, there is. Everything will draw to it and around it, but nothing can stick to its mirror finish face upon the universe or be slowed in passage around it to somewhere else via the superconducting tunneling funnel of its horizon.Reply
Nothing escapes it because, contrarily, nothing enters it passing that field of pure monopolar force . . . nothing can enter it, or resist being levitatingly (sic) funneled to some infinite elsewhere (lost in space and time) by way of that superconducting pseudo-surface hologram of a horizon.
I previously put the infinity inside the outside bounds of a black hole but realized that simply the access was all that there had to be (was all that was needed). That in a black hole -- an EWF magnetic monopole point singularity -- I was probably not dealing in anything like an emptiness but dealing in a field of purest hardest magnetic force (one that would make a neutron star or a magnetar mush) in the universe. A "supergravity singularity" couldn't exist because it couldn't possibly end up hard! cold! and elementally quantum in any way in any case! -
Atlan0001 Oops! I think I may be wrong again when it comes to the string gravity / anti-gravity element. It is roped into the picture by the strength of the magnetic monopole point singularity, producing a 'Gordian Knot' of enhanced gravity / antigravity to a superstring status I said could not exist. The combine of force and Gordian Knotted structure doesn't change a thing except to extend the power and reach of magnetic monopole point singularity and the black hole horizon superconducting funnel it is.Reply -
Atlan0001 "How did supermassive black holes get so big so fast just after the Big Bang?"Reply
You are looking through a super-version microscope, that's why.