Did dark matter help black holes grow to monster sizes in the infant cosmos?
"Finding supermassive black holes at the time when the universe was less than 1 billion years old is like finding some mammal bones among the dinosaur bones in a Jurassic sedimentary rock."
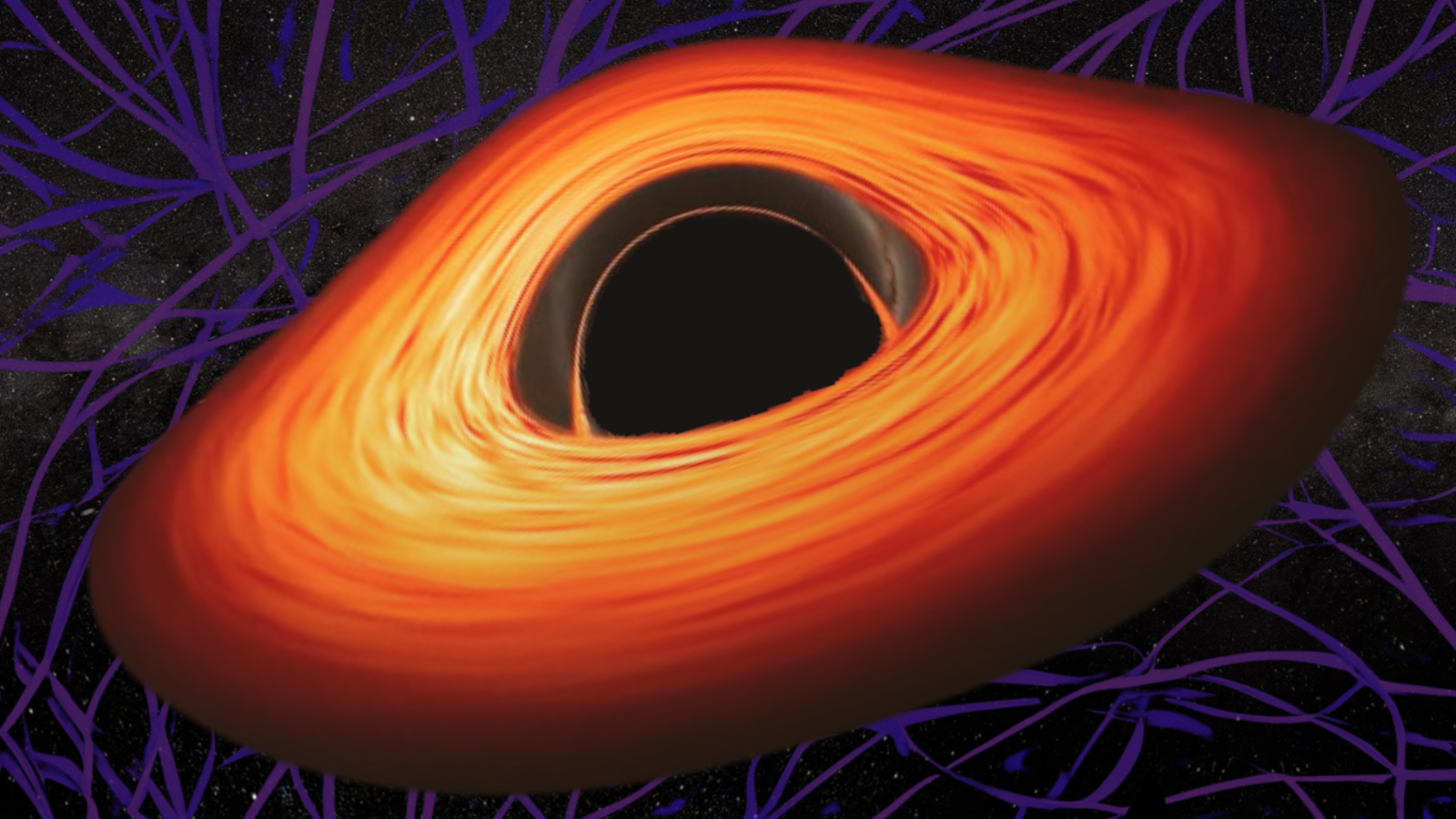
New research suggests that dark matter decay could have helped black holes grow to monstrous supermassive sizes relatively early in the infant universe. If true, this could help explain some of the most perplexing observations of the cosmos made by the James Webb Space Telescope.
Since the James Webb Space Telescope (JWST) started beaming data back to Earth in the summer of 2022, the detection of supermassive black holes with masses millions, or even billions, of times that of the sun as early as 500 million years into the life of the 13.8 billion-year-old cosmos has baffled scientists. That's because it should take at least 1 billion years for black holes to reach "supermassive status."
One hypothesis to explain how early black holes get a head start on growth suggests they are born directly from massive clouds of gas and dust. This new research, however, posits that dark matter, the universe's most mysterious substance, was a catalyst for the process.
"The formation of supermassive black holes is a mystery. Finding supermassive black holes at the time when the universe was less than 1 billion years old is like finding some mammal bones among the dinosaur bones in a Jurassic sedimentary rock," research team member Alexander Kusenko, an astrophysicist at the University of California, Los Angeles (UCLA), told Space.com. "These observations call for a very different explanation of the supermassive black hole formation.
"We found that radiation from dark matter decay could cause some large clouds of gas to collapse into supermassive black holes, solving the mystery of their origin."
Related: Dark matter could play 'matchmaker' for supermassive black holes
Solving a mystery with another mystery
Dark matter is currently considered one of the biggest outstanding mysteries in physics because, despite making up around 85% of the matter in the universe, scientists don't know what it is.
Get the Space.com Newsletter
Breaking space news, the latest updates on rocket launches, skywatching events and more!
Researchers know dark matter can't be made of the same "stuff" that makes up the atoms that comprise the ordinary matter in stars, planets, moons, asteroids and our bodies. That's because dark matter doesn't seem to interact with electromagnetic radiation (light), whereas electrons, protons and neutrons indeed do.
That lack of interaction with light also frustratingly makes dark matter effectively invisible to us, with scientists only able to infer its presence via its interaction with gravity and the effects of this interaction on ordinary matter and light.
Dark matter may not interact with light, but one of the proposed properties of this substance in some models has to do with the decay of its more unstable particles — which do release photons, the fundamental particles of light. The team thinks this radiation could be the missing piece of the supermassive black hole growth puzzle.
"Gravity can squeeze a cloud of gas and force it to collapse, so it seems possible that a million-solar-mass cloud could lead to the formation of a million-solar-mass black hole," Kusenko explained. "In reality, this does not happen because gravity works on all distance scales, and it causes small parts of a large cloud to collapse first before the whole cloud has a chance to collapse. So, instead of one giant black hole, we end up with a bunch of smaller gas clouds."
He added that if there was something to counter the action of gravity on short distances without affecting the collapse on long distances, this could spur a "direct collapse" of a huge amount of gas into a supermassive black hole. And one thing that could counter gravity is pressure.
"If the gas cloud remains hot for a long time, it cannot fragment into smaller halos because hot gas has greater pressure, strong enough to counter the pull of gravity," Kusenko continued. "This is true as long as the temperature is high enough. However, if the gas cools, pressure decreases, and gravity can prevail in many small regions, which collapse into dense objects before gravity has a chance to pull the entire cloud into a single black hole."
That cooling occurs because though the vast majority of the gas in the early universe consisted of hydrogen atoms; stars hadn't had a chance to forge heavier elements yet and disperse them with supernova explosions. Most of these hydrogen atoms would bounce off each other endlessly like billiard balls unless they were bonded into a molecule with rotational energy levels that can be excited by atomic collision.
"The excited molecule can then radiate away the energy and return to its initial state, ready for another interaction with a hydrogen atom. The hydrogen molecules become cooling agents as they absorb thermal energy and radiate it away. So, the more molecular hydrogen, the faster the cooling is," Kusenko added. "Dark matter particles can decay, producing radiation, which can dissociate [or break down] the molecules of hydrogen."
Thus, radiation from decaying dark matter could grant massive clouds of gas in the early universe the time to collapse and birth the first supermassive black holes.
"If that happens, direct collapse of hot gas into supermassive black holes becomes possible," Kusenko added.
Should this prove to be the case, what, if anything, does it tell us about dark matter itself?
"There are two possibilities: either dark matter particles can decay very slowly, or dark matter may contain a tiny component which decays fast, while the rest of dark matter is stable," Kusenko said. "In either case, the properties of radiation needed for making black holes tell us the mass of the decaying dark matter particles. This can help discover or rule out this scenario.
The team's research was published on Aug. 27 in the journal Physical Review Letters.
Join our Space Forums to keep talking space on the latest missions, night sky and more! And if you have a news tip, correction or comment, let us know at: community@space.com.
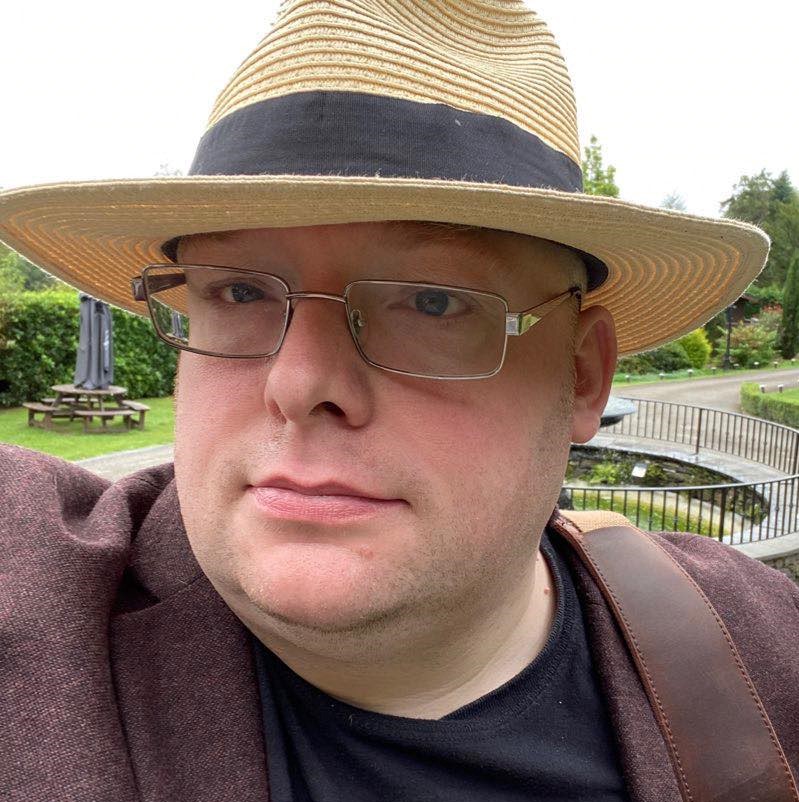
Robert Lea is a science journalist in the U.K. whose articles have been published in Physics World, New Scientist, Astronomy Magazine, All About Space, Newsweek and ZME Science. He also writes about science communication for Elsevier and the European Journal of Physics. Rob holds a bachelor of science degree in physics and astronomy from the U.K.’s Open University. Follow him on Twitter @sciencef1rst.
NASA spacecraft spots monster black hole bursting with X-rays 'releasing a hundred times more energy than we have seen elsewhere'
Could we use black holes to power future human civilizations? 'There is no limitation to extracting the enormous energy from a rotating black hole'
-
Unclear Engineer And so the BBT gets another "free parameter" to tune it to observations. This parameter is a "special type" of dark matter that is different from the dark matter that exists today. This special type "decays" and emits photons that directly interact with hydrogen molecules.Reply
Of course, regular matter that decayed on the same time scale could also do the "prevention of cooling" that is hypothesized. But, according to the BBT, no regular matter other than hydrogen and helium (and maybe a bit of lithium) had evolved yet when this effect is "needed" by the BBT.
But, that raises even more questions about how some additional type of "dark matter" that can decay at the needed time and rate could have been "made" by the BBT when regular matter was restricted to just the lightest isotopes.
If you can just make up new stuff and put it into a theory, you can make the theory do anything - except have credibility. With 95% of the BBT now being stuff that is not detectable or provable, there is a real credibility problem.
The BBT seems to be group think that is stuck on the concept of backwards extrapolating the apparent expansion of the universe at the current time to show that the universe started as a tiny, if not necessarily infinitesimal point in space.
I have to wonder what people could come up with as competing theories if they abandoned the goal of proving that everything really could have started at a tiny point-like volume of nearly infinite density and energy level.