
Euclid telescope: A scientist tells us of his quest to understand the nature of dark matter and dark energy
Euclid is the next big step forward in our quest to try to understand the universe.
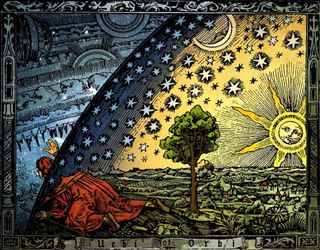
This article was originally published at The Conversation. The publication contributed the article to Space.com's Expert Voices: Op-Ed & Insights.
Henk Hoekstra is a professor in observational cosmology at Leiden University.
On July 1st 2023, Euclid, a unique European space telescope was launched from Cape Canaveral. The launch was undoubtedly the highlight of my career as an astronomer, but witnessing the result of years of work being put on a rocket is not for the faint of heart. Following a perfect launch, Euclid swiftly arrived to its planned orbit, about 1.5 million km aways from Earth. From this distant vantage point, it has started sending back sharp images that will cover nearly one-third of the sky by the end of this decade.
Euclid is the next big step forward in our quest to try to understand the universe. Over the past century we have made tremendous progress. We have learned that the fusion of hydrogen into helium powers stars like our Sun, while most of the atoms in our bodies were forged in the cores of stars that have since exploded. We discovered that the Galaxy is one of many galaxies that trace enormous foam-like structures that permeate the cosmos.
We now know that the Universe started about 13.6 billion years ago with a "Big Bang" and has been expanding ever since.
Related: Euclid 'dark universe' telescope gets de-iced from a million miles away
Probing the universe’s black box
These are major achievements, but as we learned more, it also became clear that there is much that we do not understand. For instance, most of the mass is believed to be “dark matter”, a new form of matter that is not explained by the otherwise highly successful standard model of particle physics. The gravitational pull of all this matter should slow down the expansion of the universe, but about 25 years ago we found that it is actually speeding up. This requires an even more mysterious component. To reflect our ignorance – to date, no good physical explanation exists – we refer to it as “dark energy”. Combined, dark matter and dark energy make up 95% of the universe, but we do not understand their nature.
Get the Space.com Newsletter
Breaking space news, the latest updates on rocket launches, skywatching events and more!
What we do know is that both dark components influence how large structures can form. The gravity from dark matter helps to pull together matter into galaxies or even larger objects. In contrast, dark energy pushes things apart, thus effectively counteracting the gravitational pull. The balance between the two evolves as the universe expands, with dark energy becoming ever more dominant. The details depend on the nature of the dark components, and comparison with observations allows us to distinguish between different theories. This is the main reason why Euclid was launched. It will map how the matter is distributed, and how this evolved over time. These measurements can provide the much-needed guidance that will lead to a better understanding of the dark side of the universe.
But how can we study the distribution of matter, if most of it is invisible dark matter? Fortunately, nature has provided a convenient way forward: Einstein’s theory of general relativity tells us that matter curves the space around it. Clumps of dark matter reveal their presence by distorting the shapes of more distant galaxies, just like waves on the surface of a swimming pool distort the pattern of tiles on the bottom.
Gravitational lensing and its clues
Given the similarity with regular optical lenses – the physics is different, but the math is the same – the bending of light rays by matter is referred to as gravitational lensing. In rare cases the bending is so strong that multiple images of the same galaxy can be observed. Most of the time, however, the effect is more subtle, ever so slightly changing the shapes of distant galaxies. Nonetheless, if we average measurements for large numbers of galaxies, we can uncover patterns in their orientations that have been imprinted by the intervening distribution of matter, both regular and dark.
This “weak lensing” signal may not be that spectacular, but it does provide us with a direct way to map the distribution of matter in the universe, especially when combined with distances to the galaxies for which the shapes were measured. The potential of this technique was recognized in the early nineties, but it was also clear that the measurements would be challenging. Turbulence in the atmosphere blurs our view of the faint, small, distant galaxies that we want to use, while imperfections in the telescope optics inevitably change the observed shapes of galaxies. Hence, the astronomical community was skeptical about the technical feasibility. This was the situation when I started my PhD in 1995, when I embarked on a journey to prove them wrong.
Over the years, using ever larger data sets collected with ground-based telescopes, we discovered and solved new problems. Basing myself on observations from the Hubble Space Telescope launched in 1990, my thesis work had already shown partially measuring shapes is far easier from space. However, until the arrival of Euclid, space telescopes could only observe tiny patches of sky: the James Webb Space Telescope (JWST), launched in 2021, sees the equivalent of a grain of sand at arm’s length. However, to really test the nature of dark energy we need to cover 6 million times more area. This is what led to Euclid, a unique telescope, designed to provide sharp images for 1.5 billion galaxies, as well as distance information to these. As figure 2 shows, in a single shot we observe an area larger than the full Moon.
These data are complemented by precise distances for about 25 million galaxies to map the distribution of distant galaxies in great detail.
Cosmology coordinator for Euclid
When I started my journey into this research field, dark energy had not been discovered, while few believed weak lensing would be a major tool to study the distribution of matter. How things have changed. The launch of Euclid is arguably the most spectacular demonstration of this. Since 2011 - when the project was still being considered by the European Space Agency (ESA) as part of its Cosmic Vision program - I have been as one of Euclid’s cosmology coordinators. This means I was responsible for establishing the main characteristics of the mission, in particular those pertaining to weak gravitational lensing. This included specifying how sharp the images should be, and how well we need to measure the shapes of galaxies. The work also involved frequent interactions with the European Space Agency (ESA) to clarify the science objectives and to figure out how to deal with new insights.
Thanks to hard work by a large team of engineers and scientists, we managed to overcome the many technical hurdles. We continued our collaboration through a pandemic, only to lose our intended rocket because of the Russian invasion of Ukraine - Euclid was planned to launch on a Soyuz rocket. Remarkably, ESA quickly found a solution: a launch on a Falcon 9 by SpaceX. As a result, I found myself in Florida to witness what was arguably the culmination of all my research so far.
Euclid’s obstacle course
It has been a rollercoaster ride since. The first images taken in July were noisier than anticipated, due to sunlight that seeped into the camera. This would have been a serious problem, but the most likely culprit – a protruding thruster that reflected sunlight onto the back of the sunshield – was quickly identified, as was the solution. By rotating the spacecraft ever so slightly, the thruster could be placed in the shadow of the satellite. This, however, meant a complete overhaul of the planning of the survey.
The problems did not stop there. Radiation from the sun continuously pushes Euclid around a bit, which is compensated using thrusters that keep the telescope completely stable. Only then can we take the sharp pictures we need. However, energetic particles from the Sun interfered with the stabilizing system, causing the telescope to shake a little. This was solved with a software update. Most recently, the build-up of ice inside the telescope caused concern, but that problem was also successfully tackled.
To provide the world a sense of its potential, a few “early release observations” of photogenic objects were issued in November. The one closest to my research is that of the Perseus cluster of galaxies (Figure 1). In addition to the large yellowish galaxies, which are part of this massive clump of matter, Euclid provides detailed images of another 50,000 galaxies. This level of detail is what I need for my research, but so far I only have 800 out of 25,000 such images! This has started: on February 15th 2024, Euclid started its main survey and in the next 2200 days it will keep photographing the sky. This vast amount of data will be a treasure trove for astronomers – and the whole world – for years to come. For instance, we can study in detail the structure of hundreds of nearby galaxies, such as IC 342 (Figure 3). These images are just a teaser of what the future will bring.
This article is the result of The Conversation’s collaboration with Horizon, the EU research and innovation magazine. In December, the authors published an interview with the magazine.
Read the original article at The Conversation.
Join our Space Forums to keep talking space on the latest missions, night sky and more! And if you have a news tip, correction or comment, let us know at: community@space.com.
I am an observational cosmologist that aims to shed light on the nature of dark matter and dark energy—the main ingredients of the Universe we seem to lack a good explanation for. To explore the dark side of the universe I use the bending of light rays by matter, a technique called weak gravitational lensing. Extracting the information from large imaging surveys is challenging, requiring careful calibration and corrections for instrumental effects. The inference of parameters poses challenges of its own. I have contributed to all aspects of the analysis chain thanks to leading roles in a number of ground-based projects. Now I am embarking on the next step in this journey: the harvesting of the vast and rich data provided by Euclid, an ESA mission to explore the dark universe. I coordinate the work on weak gravitational lensing for this large international project. Finally, I engage with the general public through lectures and collaborations with leading artists.