'Hawking radiation' may be erasing black holes. Watching it happen could reveal new physics.
Primordial black holes may be exploding throughout the universe. If we can catch them in the act, it could pave the way to new physics, a study suggests.
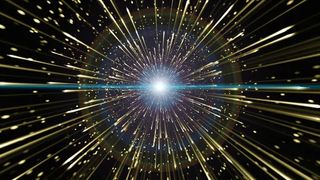
Primordial black holes (PBHs), which are thought to have formed right after the Big Bang, may be heating up and exploding throughout the universe.
These black hole explosions, powered by Hawking radiation — a quantum process where black holes generate particles from the vacuum due to their intense gravitational fields — could be detected by upcoming telescopes, physicists suggest in a new study. And, once spotted, these exotic explosions could reveal whether our universe contains previously undiscovered particles.
Black holes from the dawn of time
There's already plenty of evidence for the existence of black holes ranging from a few times the mass of the sun to billions of times the sun's mass. These black holes have been directly detected through the gravitational waves they emit during the mergers that help them grow. Some black holes, such as the Milky Way's Sagittarius A*, have even been directly imaged as "shadows" by the Event Horizon Telescope.
PBHs, first proposed by Yakov Zeldovich and Igor Novikov in 1967, are thought to have formed within the first fractions of a second after the Big Bang and may have been as small as subatomic particles, according to NASA. Unlike their larger counterparts, which form from the collapse of massive stars and galaxies, PBHs might have emerged from the collapse of ultradense regions in the extremely hot "primeval soup" of particles in the early universe.
If they exist, these compact objects could provide a natural explanation for dark matter, the invisible entity that makes up about 85% of the matter in the universe. However, PBHs remain elusive. Their theoretical existence is supported by a combination of cosmological models, but they have yet to be directly observed.
The Hawking radiation effect
One of the most interesting aspects of PBHs is their connection to Hawking radiation. According to quantum theory, black holes aren't completely "black"; they can emit radiation and slowly lose mass through a process first theorized by Stephen Hawking. This emission, known as Hawking radiation, occurs when virtual particle pairs pop in and out of the vacuum of space near a black hole's edge — its "event horizon." While these pairs normally annihilate each other, if one falls into the black hole, the other particle can escape as radiation. Over time, this leads to the black hole's gradual evaporation.
Get the Space.com Newsletter
Breaking space news, the latest updates on rocket launches, skywatching events and more!
"For black holes with masses larger than a few times that of the Sun, Hawking radiation is nearly undetectable," Marco Calzà, a theoretical physicist at the University of Coimbra in Portugal and co-author of the study, told Live Science in an email. "But lighter black holes — such as PBHs — would be much hotter and emit far more radiation, potentially allowing us to detect this process. This radiation can include a variety of particles, from photons to electrons to neutrinos."
As the PBH evaporates, it loses mass, becoming hotter and emitting more radiation in a feedback loop. Eventually, the black hole should explode in a powerful burst of radiation — a process that existing gamma-ray and neutrino telescopes are actively searching for. Although no definitive PBH explosions have been detected yet, the new study suggests these rare events could be the key to unlocking new physics.
Probing the final moments of a PBH
In their recent study, published in the Journal of High Energy Physics, Calzà and study co-author João G. Rosa, also a theoretical physicist at the University of Coimbra, introduced innovative methods for studying PBHs during their final stages of evaporation. By analyzing the properties of their Hawking radiation, the duo developed tools to estimate a PBH's mass and spin.
"Tracking a PBH's mass and spin as it evaporates could provide valuable clues about its formation and evolution," Rosa told Live Science in an email.
Their work has significant implications for fundamental physics. In a previous study, Rosa, Calzà and collaborator John March-Russell of the University of Oxford explored how string theory — an attempt to unify the fundamental forces of nature within a single quantum theory — could affect an evaporating PBH. String theory predicts the existence of numerous low-mass particles called axions, which have no intrinsic spin. Their research suggested that axion emission could actually spin up a PBH, contrary to Hawking's predictions.
"A spinning PBH would provide compelling evidence for these exotic axions, potentially revolutionizing our understanding of particle physics," Calzà said.
Furthermore, the study suggests that analyzing the evolution of a PBH's mass and spin in its final moments could reveal the existence of other new particles. By tracking the spectrum of Hawking radiation, scientists might be able to distinguish between high-energy particle physics models. Neutrino telescopes, such as IceCube, could even help uncover these new particles as PBHs explode in space.
"If we can catch just one exploding PBH and measure its Hawking radiation, we could learn a tremendous amount about new particles and potentially guide the design of future particle accelerators," Rosa said.
Although no exploding PBH has been detected yet, the tools and methods developed by Calzà and Rosa's team could pave the way for future discoveries. The researchers emphasized that dedicated experiments may not be necessary, as several new gamma-ray and neutrino telescopes with unprecedented sensitivity are already in development.
"Upcoming telescopes could easily spot one if it explodes nearby. If we're lucky enough to detect an exploding PBH, it could change everything we know about the fundamental laws of nature," Rosa said.
Join our Space Forums to keep talking space on the latest missions, night sky and more! And if you have a news tip, correction or comment, let us know at: community@space.com.
Andrey got his B.Sc. and M.Sc. degrees in elementary particle physics from Novosibirsk State University in Russia, and a Ph.D. in string theory from the Weizmann Institute of Science in Israel. He works as a science writer, specializing in physics, space, and technology. His articles have been published in Elements, N+1, and AdvancedScienceNews.
-
Unclear Engineer The whole concept of Hawking Radiation eludes me. As most do, this article says:Reply
"This emission, known as Hawking radiation, occurs when virtual particle pairs pop in and out of the vacuum of space near a black hole's edge — its "event horizon." While these pairs normally annihilate each other, if one falls into the black hole, the other particle can escape as radiation. Over time, this leads to the black hole's gradual evaporation."
First, if mass is created from energy at an event horizon, it will be matter plus equivalent antimatter. If the matter falls into the event horizon, it will add to the mass. If the antimatter falls in, it will increase mass, at least until it meets some matter inside the event horizon and goes back to pure energy. Assuming equal amounts of each go into the black hole's event horizon, how exactly does this decrease he mass of he black hole?
And, in addition, how does the particle that is not initially aimed at the black hole's event horizon ultimately escape from the event horizon? Even if it was initially going radially outward, it would need to be going at the speed of light to ultimately escape, and matter doesn't do that, although it may get close if it is subatomic. For particles moving at some angle to the radial direction, wouldn't their (undisturbed) orbit have a low point ("peri-what?) inside the event horizon? How would they get out, again? I assume that they would emit Cherenkov radiation when passing through any matter inside the event horizon, but that should not be able to escape the event horizon, either.
So I am seeing means for the black hole mass to increase, but not decrease.
Does somebody have a better explanation of how spontaneous matter creation on the event horizon can decrease black hole mass?
And, even assuming that black hole mass does decrease with time, why would it "explode". If the mass within the black hole goes to anything like a singularity or non-singularity tiny volume, the decrease in mass would make its event horizon smaller, but that event horizon would not become so small that any of the mass inside pokes through it and could escape. much less "explode". Think of a neutron star that is barely not a black hole. It does not explode because it is not inside an event horizon. If matter really can crush down more densely than a neutron star, then how does it ever get outside its own event horizon? It could not be a singularity and do that. It could not even be close to a singularity and do that.
So, what is the better explanation? Bill's bar tender wants to know, too.