
Where did the universe's magnetic fields come from?
Magnetic fields are everywhere in the universe. But where do they come from?
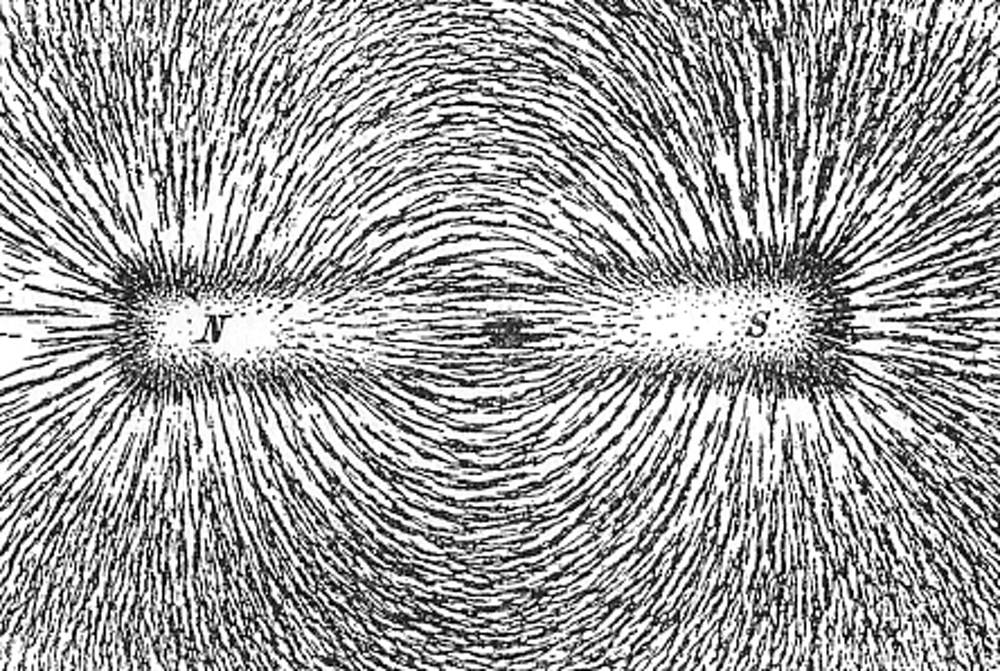
How the universe got its large magnetic fields has remained one of the stickiest outstanding problems in astrophysics. Now, researchers have proposed a novel solution: a giant "dust battery" operating when the first stars appeared.
Magnetic fields are everywhere in the universe. Of course, there's Earth's magnetic field, which deflects dangerous cosmic radiation, wiggles our compasses, and guides flocks of migrating birds. But other planets and stars have magnetic fields, too, and the magnetic fields of Jupiter and the sun are more powerful than Earth's.
Even the entire Milky Way galaxy has its own magnetic field. It's about a million times weaker than Earth's, but it stretches across tens of thousands of light-years, spanning the entire galaxy. Astronomers know of even larger magnetic fields, some of which fill entire galaxy clusters that can reach a few million light-years across.
So where do these gigantic magnetic fields come from? Even though they are relatively weak, they are incredibly large. So whatever created them must have come from suitably energetic, large-scale sources. Over the decades, astronomers have proposed a number of mechanisms, most of which rely on a dynamo process that takes weak "seed" fields and amplifies them to their present-day values.
Related: Earliest magnetic galaxy ever detected offers clues about Milky Way history
But that just pushes the goalpost back even further. Where do the weak seed fields come from in the first place?
In a paper submitted to The Astrophysical Journal in October, researchers proposed a novel solution. Their scenario starts in the cosmic dawn, when the universe was only a few hundred million years old and the first stars and galaxies were beginning to shine. After those first stars died, they left behind bits of heavier elements, which found each other in interstellar space to become the first grains of dust.
Get the Space.com Newsletter
Breaking space news, the latest updates on rocket launches, skywatching events and more!
These dust grains were generally electrically charged through bombardment with radiation and friction with each other. When the second generation of stars lit up, their intense light shone through all the gas and dust surrounding them. If these stars were powerful enough, their radiation could literally push on the dust grains, causing them to move through the rest of the gas. These moving, electrically charged dust grains would create a weak-but-wide-scale electrical current, like a copper wire 1,000 light-years across.
Because the filtering of radiation through the interstellar gas wouldn't be perfectly uniform, the moving dust grains would tend to clump in some spots and disperse in others. This would create differences in the amount of electrical current from place to place, which, through the laws of electromagnetism, would naturally give rise to a magnetic field.
In the new study, the researchers found that this magnetic field would be incredibly weak — roughly a billionth the strength of Earth's magnetic field. But it would be large enough that other astrophysical processes, like mixing and dynamo amplification, could latch on to that seed field and generate the magnetic fields we see today.
This is only a hypothesis, however. The researchers concluded their work with a recipe to include this mechanism in simulations of the evolution of galaxies and their magnetic fields. That is a crucial step in comparing the full magnetic fields predicted by this theory with the ones we see in the actual universe. We can't rewind the clock to see what the universe's magnetic fields were like long ago, but we can use ideas like this to attempt to reconstruct the past.
Join our Space Forums to keep talking space on the latest missions, night sky and more! And if you have a news tip, correction or comment, let us know at: community@space.com.
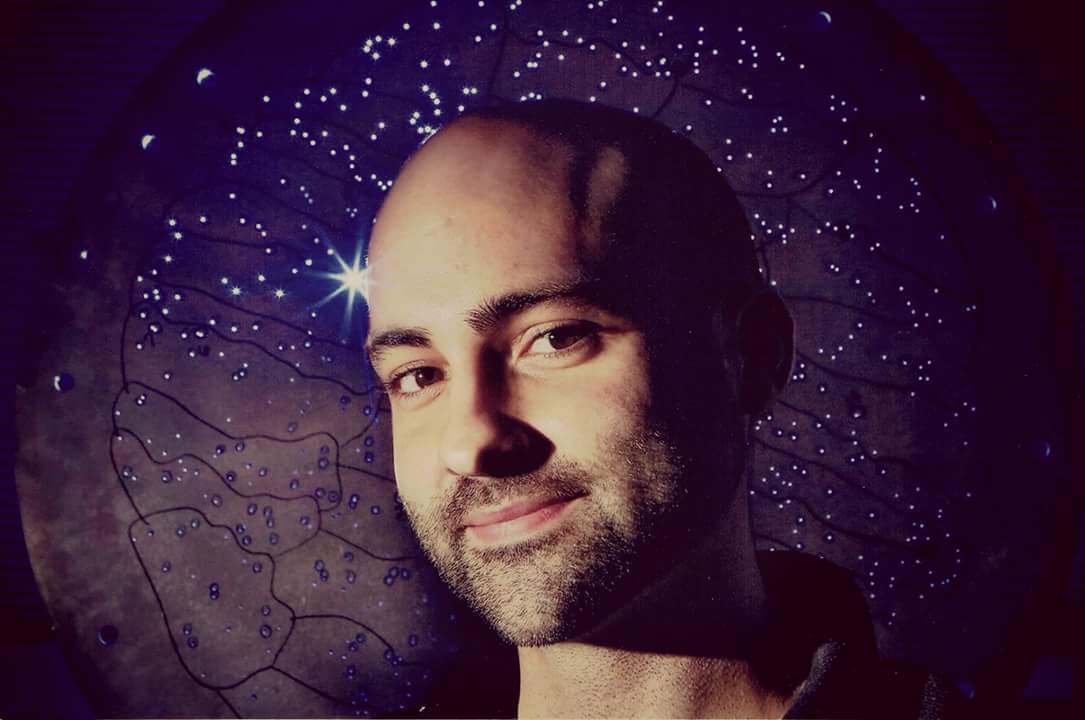
Paul M. Sutter is an astrophysicist at SUNY Stony Brook and the Flatiron Institute in New York City. Paul received his PhD in Physics from the University of Illinois at Urbana-Champaign in 2011, and spent three years at the Paris Institute of Astrophysics, followed by a research fellowship in Trieste, Italy, His research focuses on many diverse topics, from the emptiest regions of the universe to the earliest moments of the Big Bang to the hunt for the first stars. As an "Agent to the Stars," Paul has passionately engaged the public in science outreach for several years. He is the host of the popular "Ask a Spaceman!" podcast, author of "Your Place in the Universe" and "How to Die in Space" and he frequently appears on TV — including on The Weather Channel, for which he serves as Official Space Specialist.
-
Classical Motion It comes from charge. Charge is 1/2 E and 1/2 M. And unlike E, M can be manufactured beyond what charge gives us. M is analog, while E is digital.Reply -
orsobubu
I wonder whether it is reasonable to ask questions such as the ultimate origin of the universal magnetic field, if quantum electrodynamics theory is still based on idealistic and exclusively mathematical absurdities such as virtual particles and messenger photons.Classical Motion said:It comes from charge. Charge is 1/2 E and 1/2 M. And unlike E, M can be manufactured beyond what charge gives us. M is analog, while E is digital. -
Atlan0001 A magnetic field has nothing to do with the speed of light, so a magnetic bubble of field centering upon a galaxy realized to be dealing in the entire flatland disk of the galaxy, above and below it, is as unitary as any other magnetic field of physics. It exists over and across space and over and across time, but being unitary, not over SPACETIME.Reply
SPACETIME has too few dimensions (just four) . . . and/or too many dimensions (four more than zero) . . . for a unitary magnetic field. -
billslugg There is no such thing as a magnetic field, it is simply the electric field adjusted for relativity when any charge is moving relative to the observer. The "reality" of the magnetic field is no more "real" than the contour lines on a map.Reply
The fact we can detect a "magnetic field" of the universe tells us there is some charge out there and it is moving. -
Atlan0001
It is no more limited to SPACETIME than a quantum entangling wormhole or blackhole.billslugg said:There is no such thing as a magnetic field, it is simply the electric field adjusted for relativity when any charge is moving relative to the observer. The "reality" of the magnetic field is no more "real" than the contour lines on a map.
The fact we can detect a "magnetic field" of the universe tells us there is some charge out there and it is moving. -
Atlan0001 To widen the meaning, it is no more limited to SPACETIME's astronomical slows (the slowness) of the speed of light than a quantum entangling wormhole or blackhole. A galaxy is in the 'hole' (the quantum 'hole' if you will) of a magnetic field.Reply -
Atlan0001 Ad#7:Reply
Which is probably why it can 'hold' together throughout (maybe without need of dark matter) without flying apart. -
Classical Motion The E is a one direction isotropic field. It’s linear. And it’s self repulsive. Divergent.Reply
The M is bi-directional field, an angular field. And it’s closed. Easily converged. With at least one area of convergence. But can have multiple “poles”. Can be put in series. An attractive series.
These are very different dynamics. And why, you don’t see the M until alignment. But it’s always there. Just like E.
Moving a charge does not convert the fields. It just aligns them.
It takes an alignment flux to detect the M field. A flux of charge alignment. And current is an aligned flux. Some metals can be induced and hold that alignment. Some can be molded with alignment. -
Classical Motion Most think that charge, e, is a spec of electrical potential. Don’t do that. Think of charge as a line of current. This is particle current, not electrical electron current.Reply
Think of e as a current loop. And every-time it rotates once, it’s one e of current.
The speed of the rotation is constant, and the only way to change that current is to change the length of the rotation. Which varies the RPM. A ground state charge has about 18 amps of current. But for a proton, the rotation is so short, the proton has about 30,000 amps. These are huge differences. In energy AND size. And RPM.
For an E potential, only a net number in the vicinity is needed. BUT for an M field, that net charge has to be aligned. If it’s not aligned, you can’t detect it. M must be aligned for superposition. E needs no alignment.
A copper wire has just a much free M dipoles as it has free charge and free e. To give the wire an E potential, only separation of free e is needed. But for the M, free alignment is necessary. A current will align the charge. And let you see M.
Ampere had it right all along. Current loops.
E is number, M is alignment. -
Atlan0001 It is either the physic of magnetic fields or a different quantum based physic altogether, to wit the strong interaction (the strong force) present in the macrocosm as well as the microcosm . . . as I've claimed before (the microcosm modeling the actual omni-directional magnitudinous extended broader and deeper entity of the macrocosm beyond the superficial local-relative observable and that entity being the model, the microcosm, back)! Look up the "Cantor set" in Wikipedia for an illustration of the breakage . . . aka the unobserved, unobservable, missing universe in all of an infinity of folds (an infinity of folded universe) in the so-called "accelerating expansion of the universe"!Reply